- Title: Helium ignited violent mergers as a unified model for normal and rapidly declining type 1a supernovae
- Authors: Pakmor, R., M. Kromer, and S. Taubenberger
- 1st author’s home institutions: Heidelberger Institut für Theoretische Studien, Heidelberg, Germany & Kavli Institute for the Physics and Mathematics of the Universe, University of Tokyo, Japan
Type Ia supernovae both created a substantial fraction of the heavy elements in the Universe (no small task!), and played the lead role in the Nobel prize-winning discovery of dark energy. Because we can figure out how bright these explosions were based on how long they take to fade away (as discussed in this astrobite), we can compare their intrinsic and observed brightnesses to determine their distances (the farther away the explosion is, the dimmer it appears). Astronomers used these bright “standard candles” to measure the expansion rate of the Universe, and revealed that dark energy is causing the Universe to expand at an accelerating rate. Given the weight of this discovery, it might surprise you to learn that we don’t actually know what Type Ia supernovae are!
What we know: Type Ia Supernovae come from stellar remnants
Okay, we aren’t 100% clueless: astronomers have some ideas, but haven’t been able to establish a single, best model. Let’s start with what we know. First off, Type Ia’s, unlike other supernovae, don’t result from the deaths of massive stars. If that were the case, we’d see them in regions of where stars recently formed since the most massive stars only live for a short time; instead, we tend to see Type Ia supernovae among older stars, for instance, in elliptical galaxies which have stopped making new stars. That means they must originate from a stellar remnant, presumably a white dwarf.
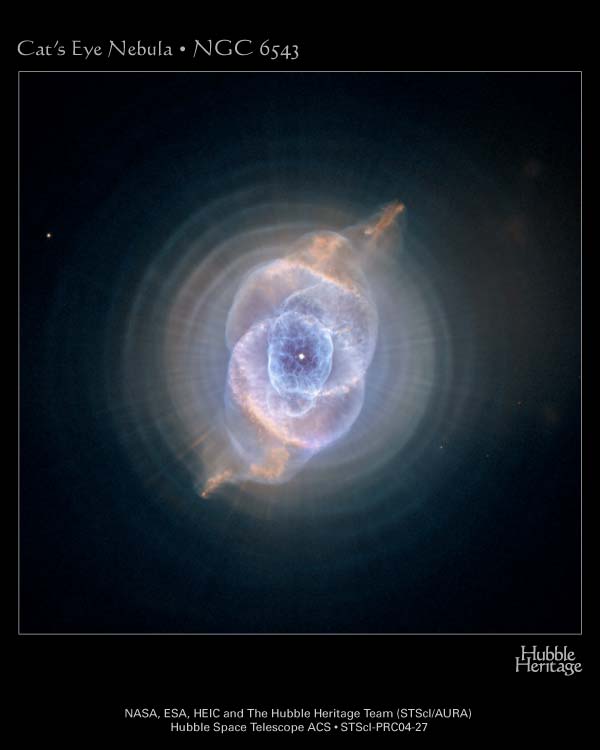
Figure 1: An example of a planetary nebula. The white dwarf appears as a tiny white dot in the center. Photo credit NASA, ESA, HEIC, and The Hubble Heritage Team (STSci/AURA).
White dwarfs & degeneracy
White dwarfs are the cores of low mass stars, left behind after the stars shed their outer layers to create “planetary nebulae”, like the Cat’s Eye Nebula in Figure 1. (Note that planetary nebulae have absolutely nothing to do with planets–see Susanna’s astrobite for a more detailed discussion of one.) Most white dwarfs are essentially giant diamonds, composed of carbon and some oxygen which crystallize inside the remnant. They are so dense that electron degeneracy pressure, not thermal pressure, holds them up. According to quantum mechanics, when electrons are squeezed into a very small space, they move very quickly. Degenerate electrons zoom around rapidly enough to support the white dwarf against the crush of gravity, much like fast-moving particles in a hot gas. However, unlike normal gas pressure, electron degeneracy pressure doesn’t depend on temperature. Because of this, it’s basically impossible to make lone white dwarf do anything exciting: it will sit around cooling off forever. To explode in a brilliant supernova, the white dwarf needs a companion.
It’s all about the companion
A nearby companion can ignite carbon fusion in the white dwarf by dumping mass onto it. The nature of that companion, and how exactly the mass transfer starts up carbon fusion, is the big question here. Originally, astronomers thought white dwarf supernova progenitors were paired with red giants, a late evolutionary stage of low mass stars. Red giants are so bloated that they can barely hold onto their hydrogen outer layers, making it easy for the white dwarf companion to steal material. As mass is loaded onto the white dwarf, the excess weight compresses it and causes it to heat up. (Note that although thermal pressure is not holding up the white dwarf up, its temperature will still increase as its pressure and density increase.) If the core gets hot enough, carbon can start to fuse into heavier elements, releasing energy in the process. Normally, a star would react by expanding and cooling, slowing the reaction rate. But because electron degeneracy pressure is independent of temperature, it’s as if the white dwarf’s internal thermostat is broken. Once fusion begins, it takes off and obliterates the white dwarf within seconds: a Type Ia supernova.
Unfortunately, this simple model doesn’t quite work. For one, most stellar population models don’t predict as many white dwarf/red giant pairs as we would need to match the observed rate of supernova explosions. Moreover, if a red giant assisted in the explosion, we should see it among the debris, but several Type Ia supernova remnants appear to lack remaining companions (like the one in this astrobite). Pakmor et al. consider an alternative: the double-degenerate pair.
White dwarf meets white dwarf
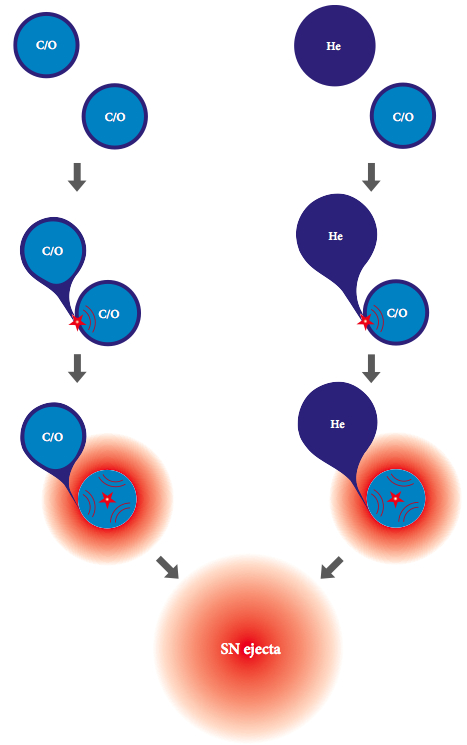
Figure 2: Pakmor et al.'s proposed mechanism for Type Ia supernovae. On the left, a carbon/oxygen white dwarf accretes helium from a second carbon/oxygen white dwarf; on the right, a carbon/oxygen white dwarf accretes from a (lower mass) helium white dwarf. Helium is in dark blue; carbon & oxygen are in light blue.
A double white dwarf explosion addresses the problems of the white dwarf/red giant model: double-degenerate pairs are both common and would leave no trace (when one white dwarf explodes, so would the other). Pakmor et al. suggest a new explosion mechanism for the white dwarf/white dwarf pair–one that could lead towards a single model for all Type Ia supernovae.
In the Pakmor et al. model, a carbon and oxygen white dwarf grabs helium from a second, lower mass white dwarf, which can either be composed of carbon and oxygen, or of pure helium (Figure 2). (Helium white dwarfs come from very low mass stars, in which electron degeneracy pressure stops the core from contracting early on in its evolution. In these stars, the core never reaches the high temperatures needed to fuse helium into carbon.) Clumpiness in the inflowing material or a collision of the two white dwarfs sparks helium fusion in the thin layer around the primary white dwarf. At that point there’s no turning back: the primary heats up and everything that can explode, does explode.
A unified model?
This theory nicely explains the variety of Type Ia supernova we see. Carbon and oxygen companions produce brighter supernovae that last for a long time since they have more material to fuse; helium companions produce dimmer supernovae that fade away quickly. In an extreme white dwarf/white dwarf interaction, the companion could be ripped apart by tidal forces and form a disk of material around the primary; this might explain rare, ultraluminous supernovae. If future studies support the ideas presented here, they could lead towards a better understanding of these bright, guiding lights. Until then, Type Ia supernovae remain an important unsolved mystery!
Trackbacks/Pingbacks