Title: Failed supernovae as a natural explanation for the binary black hole mass distribution
Authors: P. Disberg and G. Nelemans
First Authors’ Institution: Department of Astrophysics/IMAPP, Radboud University, P.O. Box 9010, 6500 GL Nijmegen, The Netherlands
Status: accepted to Astronomy & Astrophysics [open access]
The study of gravitational waves (GWs) informs us of the lives of massive stars which become binary compact objects – black holes and neutron stars. These stars live fast and die young, making young and massive stars close enough to study individually with electromagnetic observatories hard to come by. Gravitational waves – tiny ripples in spacetime detected with extremely sensitive lasers – provide signatures of these compact objects as they merge, and make for a new observational window to their predecessors’ evolution.
Studying these ripples allows GW observers to infer certain properties of the remnant black holes: their masses, spins, redshifts, and more. Comparing theory to the observed properties of all the detected compact objects allows gravitational wave paleontologists to make predictions about the lives of their progenitors, the physical processes in their evolution, and the environments in which they form.
With more and more detections, the features in the distributions of these remnant properties become more and more distinct. This gives theorists, who work on find astrophysical explanations for the observations, better information to predict the lives of the compact object progenitors. Today’s authors look at predicting the origins of the gap in the mass distribution of detected black holes between 14-22 solar masses, as shown in Figure 1, that has emerged with the current set of almost 100 GW detections made so far.
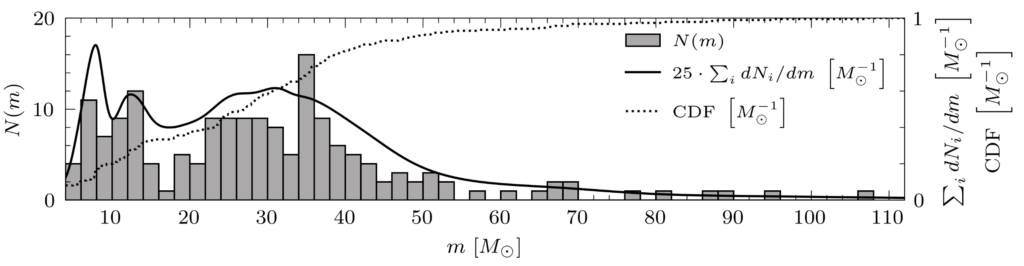
The mass of a merging compact object depends greatly on the processes its progenitor undergoes during supernova (SN). This is the stage in its life when its core, no longer undergoing fusion, reaches the maximum possible mass and collapses, before sending out a shockwave, ripping the star apart. This ejects the outer layers of the star into the cosmos; the outer mass of the star is removed, creating a glowing aura around the collapsed stellar core which itself collapses.
However, there is a caveat: sometimes this explosion is halted due to other processes in the star. This leads to an unsuccessful supernova: no ejecta, no bright explosion, and a star that simply vanishes out of the sky with all of its mass having fallen into the black hole it has created. This vanishing act creates a remnant black hole more massive than it would be if the supernova wasn’t stalled. This only occurs for stars within a certain range of masses, leaving a gap in the distribution of black hole masses we detect. The question is then, if the gapped mass distribution is created by these vanishing stars, at which masses do the supernovae succeed or fail in order to match observations? Are there enough failed supernovae to account for the shape of the mass distribution that we see?
To investigate this, the authors create a prescription for the relationship between the initial pre-supernova mass of the stars and the black hole masses that remain after the (successful or failed) supernova, as well as the rates both kinds of supernovae occur at. Previous studies simulated supernovae to find that progenitors with masses between roughly 20 and 24 solar masses have a failed supernovae, and there is a region of successful supernovae above this until 27 solar masses, where the failed SNe take over again.
Because the stars with successful supernovae in the 24-27 solar mass range eject some of their outer layers, the remnant masses from these stars are smaller than the progenitors with failed supernovae. This creates a deficit of remnants with masses from about 14-22 solar masses (i.e. the gap), as shown with the gray band in Figure 2. The vanishing stars that undergo a failed supernova at low masses seen on the left of the gap in Figure 1, leaving black holes in the 8-14 solar mass range, are dubbed the “black hole island”.
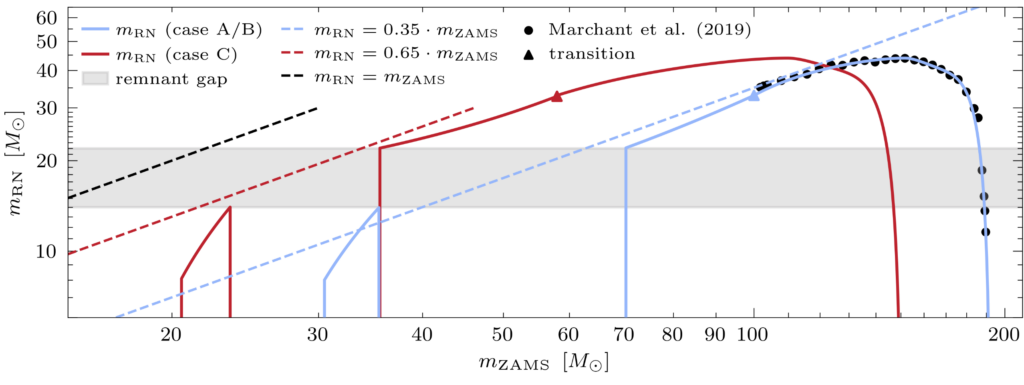
To check if the vanishing stars really do exist in their proposed mass range, the authors investigate if failed supernovae make a noticeable impact on the rate of supernovae we detect. If we see less supernovae than we would expect from how many stars we know form at the right masses, then it means there could be some invisible failed supernovae to compensate for the mismatch. The observations they consider support their models prediction that 25% of these supernovae are of the vanishing type. However,if they slightly change the mass limits where failed supernovae occur, or the number of high mass stars that form, the observations don’t support any vanishing supernovae.
Lastly, the authors take the remnant mass prescriptions from Figure 2, and use this to construct a mass distribution of BHs to compare to the observations in Figure 1. Starting with 1.2 million binary stars chosen from the entire mass range, and modeling some mass transfer between the stars in each binary, they recover the mass distributions shown in Figure 3.
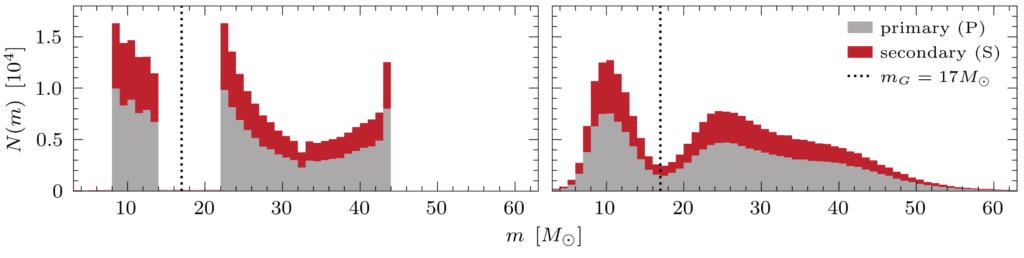
This distribution distinctly shows that this supernovae prescription creates one peak at around 10 solar masses, and a gap until around 22 solar masses. This lower peak is caused by the failed supernovae in the BH island, and so it seems like these vanishing stars would be a natural explanation for the lower number of black holes detected between 14-22 solar masses. While the observations show a larger number of high mass black holes than can be created with failed supernovae, these may come from other formation channels which allow for higher masses, or some maybe even some unknown physics not considered yet.
Vanishing stars are off to a confident start in island life, and future studies with more detailed models of the populations of these massive stars, together with direct fits of the modeled populations to the observations, could help secure their ocean home. Once we know more about how often supernovae occur, we might be able to ‘see’ the stars that disappear, so keep an eye out for the stars that disappear when your head is away in the cosmos.
Astrobite edited by Roel Lefever
Featured image credit: By invisiblepower, modified by Storm Colloms