Title: A coherent radio flash following a neutron star merger
Authors: A. Rowlinson, I. de Ruiter, R. L. C. Starling (10 additional authors)
First Author’s Institution: Anton Pannekoek Institute for Astronomy, University of Amsterdam, Netherlands
Status: Submitted to Nature [open access on arXiv]
Neutron stars (NSs) are the densest objects in the universe, excluding black holes; a teaspoon of neutron star would weigh as much as Mount Everest. The internal structure of neutron stars, while hard to directly study, can teach us much about this realm of high-density physics which we cannot recreate in laboratories on Earth. So, why don’t we smash them together and see what happens? Neutron star mergers (when two orbiting NSs collide) do take place, and we’ve observed them in the form of short gamma-ray bursts (GRBs) as well as gravitational wave events with LIGO.
What’s left over when they collide? Neutron stars have a maximum mass limit, above which they collapse into a black hole under their own weight. The specific value of this mass threshold depends on the inner workings of the NS, but it is likely around 2 solar masses, less than the total combined mass of two ~1.4 solar mass NSs colliding. While the remnant of such a merger will likely end up as a black hole, the remnant begins its life rapidly rotating and highly magnetized; the rotation and magnetic field could help counteract the self-gravity of the massive object, and the remnant could spend a small amount of time as an over-massive NS before collapsing into a black hole.
Neutron stars can emit radio waves (e.g. pulsars and magnetars) while black holes can’t, so radio observations of the newly formed object could potentially determine what the NS merger left behind. Charged particles in the interstellar medium delay the arrival of lower-frequency radio waves compared to the higher-frequency gamma rays, so as soon as a GRB is detected, radio observations of that region of the sky can be triggered to look for these characteristic NS features. Many such radio observations have been taken in the wake of GRBs, but none have been fruitful in finding radio emission. Today’s paper reports the first likely detection of a radio burst following a neutron star merger!
Pop! Goes the Radio Flash
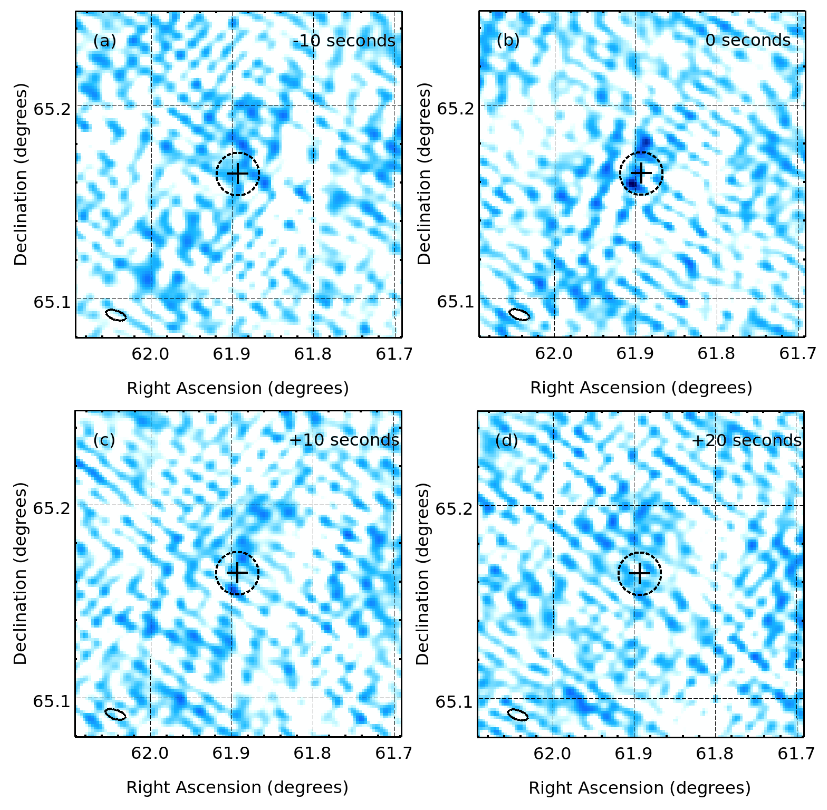
GRB 201006A was discovered with the Swift X-ray satellite on October 6th, 2020. Today’s authors used the LOFAR radio telescope array in Europe to trigger deep-field snapshot observations only 5 minutes after the GRB detection. By breaking the data into 10-second intervals and searching for emission which rises and falls on such quick timescales, the authors found a short-lived radio burst, shown in the top right panel of Figure 1.
It’s important to estimate our distance to the source in order to estimate its luminosity and to theorize about its surroundings. Distance estimates in astronomy are famously difficult, but the GRB had no optical counterpart or identifiable host galaxy, so the authors had to use the dispersion of the radio burst to estimate a distance.
This exciting discovery comes with a few caveats: the radio burst is relatively dim and reasonably offset from the position of the GRB. The radio burst has a detection significance of 5.6-sigma– the peak of the signal is 5.6 standard deviations above the average noise level. Typical transient searches may have minimum detection thresholds of 5-7 sigma, so the detection was not astoundingly bright, but the authors did their due diligence to confirm that the pulse is real and astrophysical.
The burst location is also offset from the location of the GRB by 27 arcseconds, which is roughly the size of Jupiter as seen from Earth (too small for the naked eye to distinguish). That’s a larger offset than astronomers usually like to see, but not so largely separated that it’s impossible the two are related. The authors once again performed statistical tests to show that the likelihood of finding a completely unrelated radio transient at such a similar time and place to the GRB is less than one in a million.
What are we even looking at?
Assuming that the radio flash and the GRB truly come from the same source, what can that tell us about the result of this neutron star merger? A lot of signs point away from this object being a black hole; material falling onto a black hole can produce a radio flash, but black holes are massive enough to pull all of the material in within a few seconds of formation, so we don’t expect to see radio emission from black holes after that. This radio flash was emitted almost an hour after the GRB, and other properties of the GRB also indicate that this is not likely a black hole.
Much more plausible is the possibility that we’re observing a short-lived neutron star before it collapses into a black hole. Figure 2 plots the duration and luminosity of the detected radio flash against short-lived radio bursts from neutron stars (pulsars, RRATs, and magnetars) as well as other radio transients like fast radio bursts (FRBs). The burst looks closest in nature to FRBs, which actually invoke neutron stars as a possible progenitor.
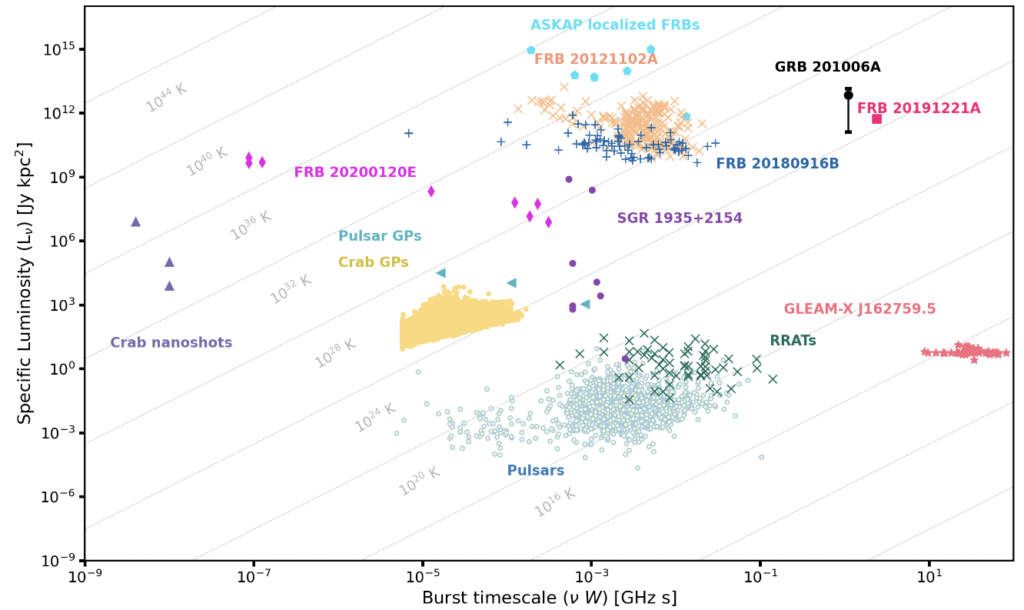
As a final tantalizing possibility, the radio flash could come from the collapse of the proto-neutron star into a black hole. The burst was emitted 48 minutes after the GRB, and we expect that the high magnetic field and rapid rotation can sustain a NS for a few hours before it loses enough energy to initiate collapse. The brightness of the radio burst is also consistent with the brightness we’d expect of a collapsing NS– this depends on a lot of assumptions about the distance to the object, the efficiency of the conversion from gravitational energy to radio emission, and many properties of neutron stars themselves. But these observations could be our first direct ‘snapshots’ of a neutron star collapsing into a black hole!
Edited by William Lamb
Featured image credit: University of Warwick/Mark Garlick