Authors: Vrutant Mehta, Jack Sullivan, Robert Fisher, Yuken Ohshiro, Hiroya Yamaguchi, Khanak Bhargava, and Sudarshan Neopane
First Author’s Institution: Department of Physics, University of Massachusetts Dartmouth, USA
Status: Submitted to MNRAS [open access]
Just like humans, there are many different ways in which a star can die. In broad terms, astronomers talk about supernovae (SNe), which are the luminous and cataclysmic deaths of stars. However, things aren’t so straight forward. Stellar deaths can be classified based on a variety of factors, including the properties of the star before it died, the explosion itself, and even the environment in which the supernova occurred.
But for today’s bite, we’re particularly interested in Type Ia SNe. These highly luminous stellar deaths occur when the compact stars known as white dwarfs (WDs) accrete matter from a companion star until their mass equals (or is close) to the Chandrasekhar Mass (Mch), the theorised maximum mass of a WD. In today’s bite we’re looking at 3C 397 (see figure 1), a galactic Type Ia supernova remnant that was analysed by a group of eager researchers hoping to better understand the ins and outs of stellar death.
RIP 3C 397, a beloved Type Ia Supernova Remnant
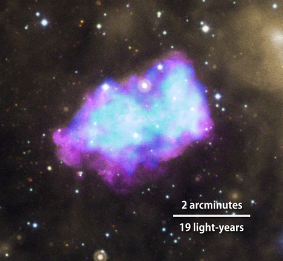
3C 397 is located in the Milky Way galaxy, and has been observed at a variety of wavelengths, but most importantly, in X-rays. X-rays are a great way to understand supernova remnants because they can reveal which elements are present in the ejecta, the stellar material that is expelled by the star as it dies. This in turn hints at the composition and explosion type of the star before it died.
Importantly, 3C 397 was observed with the X-ray space telescope Suzaku, which showed high mass ratios of Mn/Fe, Ni/Fe, and Cr/Fe, elements that are all expected to be present in large amounts in Type Ia supernova remnants. By comparing these results to models, researchers realised that the progenitor of 3C 397 must have been a near-Mch WD. In addition, XMM-Newton observations revealed that the distribution of iron-group elements in the ejecta was unevenly distributed and clumpy. While 3C 397 is classified as the remnant of a Type Ia SN, the authors of today’s paper wanted to understand even more about its tragic demise, namely, the precise explosion mechanism that took place.
Deflagrations, Detonations, and Everything in Between
For near-Mch Type Ia SNe, there are two main explosion mechanisms, known as deflagration-to-detonation transitions (DDT) or pure deflagrations. DDT Type Ia SNe occur when a slow-burning bubble rises buoyantly through the convective region of a near-Mch WD, eventually triggering a nuclear detonation which completely destroys the star in the process. Pure deflagrations also consist of a bubble that combusts and travels through the body of the WD, however, these events never trigger a detonation, permitting the bubble to reach the surface. Since only a small part of the WD is burned, the resulting supernova is dimmer and occurs over a shorter timescale compared to a DDT event.
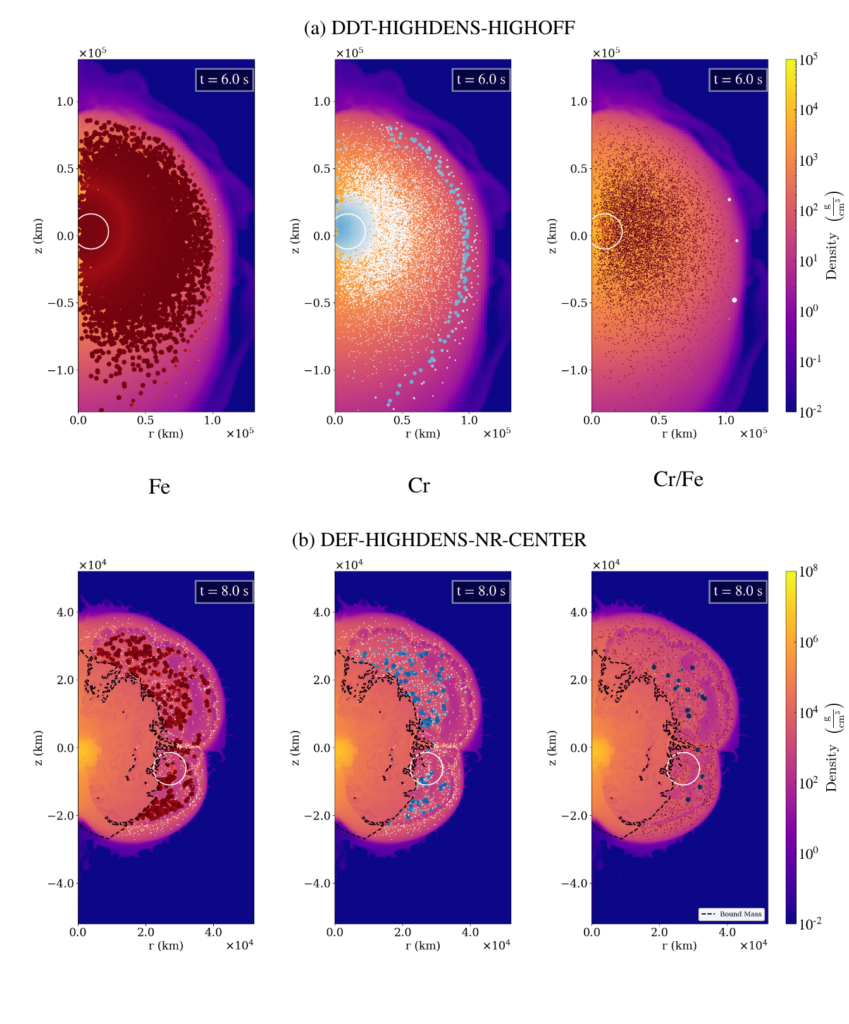
Therefore, given that 3C 397 is the remnant of a near-Mch Type Ia SN, it was most likely formed either via a DDT or pure deflagration. To understand which mechanism occurred, the authors of today’s paper conducted a suite of two-dimensional, hydrodynamical simulations varying a range of properties including the density and metallicity of the WD, and the explosion type.
The results of their simulations can be seen in Figure 2, which gives the final supernova ejecta distributions for the DDT model with a high density and large bubble offset from the centre of the WD above, and for the pure deflagration model with a high density and a small bubble offset below. From left to right, the three columns show the distributions of iron, chromium, and the ratio of chromium to iron, two important elements for near-Mch explosions.
What the authors immediately noticed was the difference between how structured the ejecta is in each case. More specifically, the DDT model is highly stratified and ordered, while the pure-deflagation model is highly asymmetric and clumpy. The authors argued that the disorder seen in their pure-deflagration simulations is an imprint of the fluid instabilities that were present in the star prior to explosion, which were maintained due to the lack of detonation. On the other hand, the structure of the DDT model is ordered since the detonation wiped away any trace of these hydrodynamical instabilities.
To end, the authors compared their simulation outputs to the real-life observations of 3C 397 from Suzaku and XMM-Newton. What they found was that the ejecta clumpiness as well as the Cr/Fe ratios were well-reproduced by the pure-deflagration model with a high central density. So while the star that created 3C 397 might be lost, it is not forgotten, at least while there are keen astronomers simulating its death and trying to understand its tragic demise.
Astrobite edited by Junellie Gonzalez Quiles
Featured image credit: NASA/Suzaku and NASA/CXC, DSS, and NASA/JPL-Caltech