Title: Radio Pulsar Sub-Populations (I) : The Curious Case of Nulling Pulsars
Authors: S. Konar, U. Deka
First Author’s Institution: National Centre for Radio Astrophysics (Pune, India)
Status: Published in the Journal of Astrophysics and Astronomy, open access on arXiv
Pulsars are some of the most extreme objects in the universe. With their incredible densities (a teaspoon would weigh as much as Mount Everest) and extreme magnetic fields (which are 12 orders of magnitude stronger than Earth’s), these objects are some of the strangest we have ever come across. Pulsars emit radiation like a lighthouse and sometimes they just turn off and do not emit at all. Today’s work explores this strange behavior of pulsars in order to try to understand what types of pulsars show this behavior and why.
The Strange World of Pulsar Emission
Pulsars are rapidly-rotating, highly-magnetized neutron stars that emit radiation along their magnetic axis which is tilted with respect to their rotation axis (see Figure 1). This causes their radiation to sweep along our sightline like a lighthouse. There are two types of pulsars: canonical pulsars (pulsars that have rotation periods between a few seconds and tens of milliseconds) and millisecond pulsars (pulsars that have been recycled with rotation periods on the order of just a few milliseconds). Most pulsars emit in the radio regime but they can also emit X-rays. Radio pulsars emit radiation which is observed as narrow emission pulses. All pulsar emission is characterized by production of matter/antimatter pairs (a process called pair production) in the magnetosphere which leads to the production of the radio emission. Sometimes that emission abruptly stops for several pulse periods. This cessation of emission is a phenomenon known as nulling.
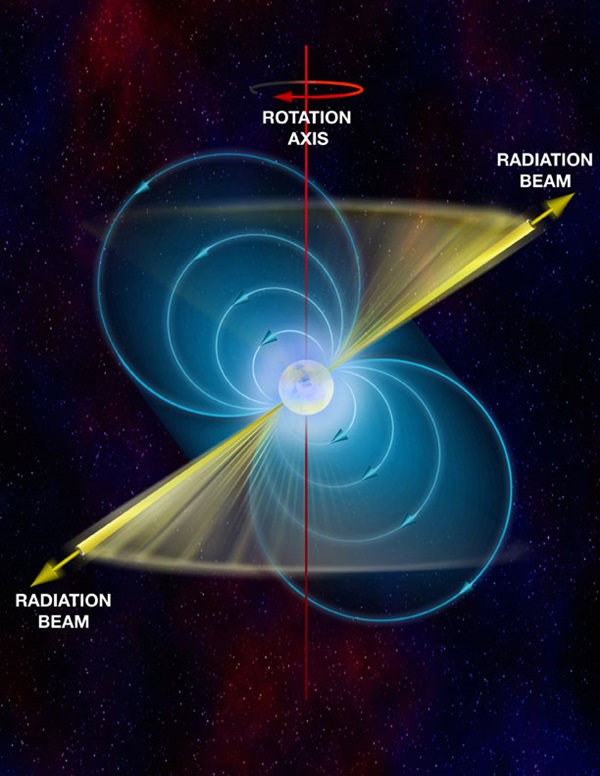
Image Credit: Credit: Bill Saxton, NRAO/AUI/NSF
Since the phenomenon’s discovery, more than 200 out of the ~2600 radio pulsars have been seen to experience nulling (~8%). Two parameters are used to characterize the phenomenon of nulling: null fraction (the total fraction of pulses without detectable emission) and null length (the duration of a nulling episode). Both of these numbers can exhibit a wide range of values; the null fraction can go from just a few to over 90%, and the null length can go from a few single pulses to a complete disappearance of the emission for years at a time.
Why Do Pulsars Null?
Nulling is thought to be caused by one of two things: intrinsic effects within the pulsar or geometric effects due to spin and rotation. With internal effects, astronomers think that nulling could be caused by lack of pair production in the magnetosphere or changes in the flow of the magnetosphere itself. On the geometric side, it is theorized that nulling is a result of the pulsar emitting regularly but simply turning away from us so we do not see the emission. In order to better understand what kind of pulsars null and why, the authors of today’s work examine the largest sample of nulling pulsars ever studied and look at the correlation (or lack thereof) between nulling fraction and various pulsar parameters such as age, and find some interesting results.
Characterizing Nulling Pulsars
Studies have been done to try to correlate the null fraction with pulsar parameters in order to better understand why some pulsars just turn off. One study analyzed 72 nulling pulsars and found the rotation period of the pulsar to be directly proportional to nulling fraction. Another found correlations between nulling and pulsar age. However, with their large sample, the authors found little to no correlation between pulsar period, surface magnetic field, age, or dispersion measure (a measure for the degree of dispersion of the emission caused by stuff between us and the pulsars), suggesting age does not play a role (see Figure 2). However, they did find a gap in the estimated nulling fraction around 40%, separating the pulsars into a population experiencing higher and lower nulling fractions. They find that there are more pulsars with lower nulling fractions (<40%) than higher ones, which could simply be an observational bias. The distributions of the intrinsic pulsar parameters (period, change in period, age, surface magnetic field, etc.) are statistically different in the two populations, but there is no correlation between nulling fraction and any intrinsic parameters.
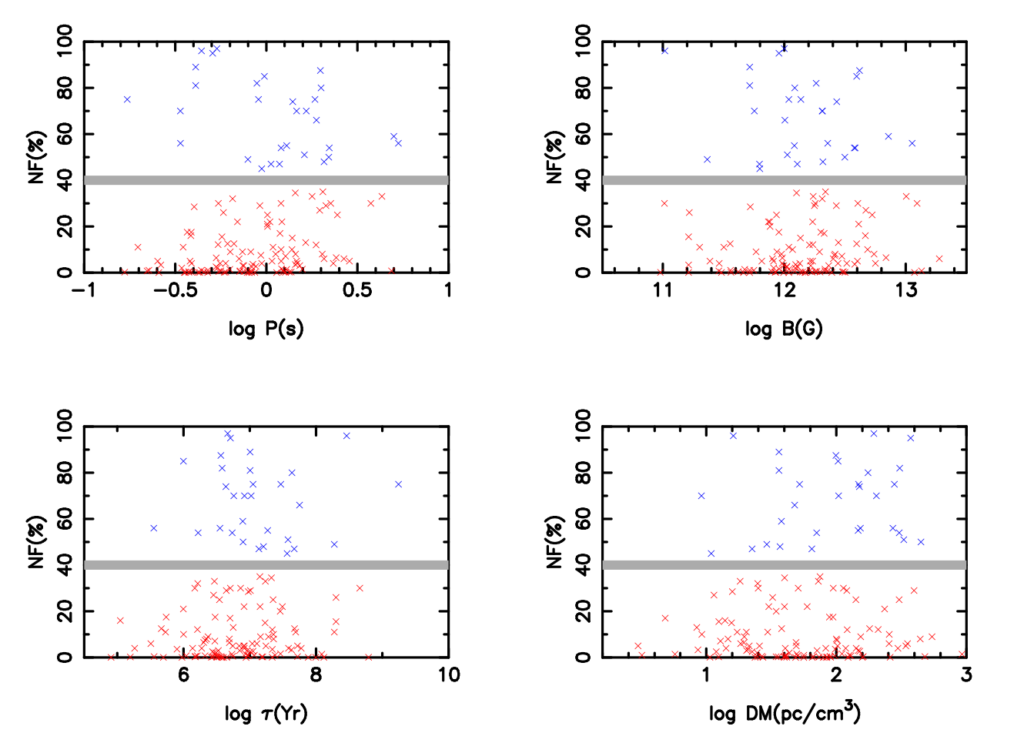
Modeling the Journey to the Pulsar Graveyard
As pulsars age, they lose energy as they emit radiation. This loss in energy causes the pulsars to slow down, or “spin down,” and they get slower and slower until they eventually get to the point where they no longer emit radiation. When this happens, they cross what is called the “death line” which is quantified using the pulsar period and change in pulsar period or the pulsar period and the magnetic field. While the authors have ruled out a definite correlation between age and nulling fraction, many believe that older pulsars, ones closer to the death line, are more likely to null.
Nulling is thought to be an intrinsic phenomenon, so scientists think there must be some relation between the emission mechanism and nulling behavior. Because the emission mechanism of pulsars is not fully understood, there are many different models to describe the process the full cessation of the radio emission. These models simulate the pulsar magnetosphere in different ways, for example, some theorizing that the magnetic field has very curved lines at the top of the star, and some using the fact that the magnetic field can be described as a dipole that is not centered in the middle of the neutron star. Each of these uses a different emission model and produces a slightly different model of where the “death line” should be. Figure 3 shows the different death lines that the models produce. The top panel shows all pulsars plotted with the different death line models (the red and blue lines) while the bottom panel shows the nulling and intermediate pulsars (pulsars that experience short nulls).
By plotting all of the known nulling pulsars with all of the death line models, it is clear that some models constrain the pulsars better than others. The authors find that the models that best constrain the pulsars are ones that involve the pulsar having very curved magnetic field lines and possessing a magnetic field that is not purely a dipole. These conclusions point to the fact that pulsars that have emission coming from primarily from the polar cap region (the top of the star) and that have extremely curved magnetic field lines are more likely to experience more nulling. The fact that some pulsars are beyond the least constraining models points to the fact that the mystery is not entirely solved.
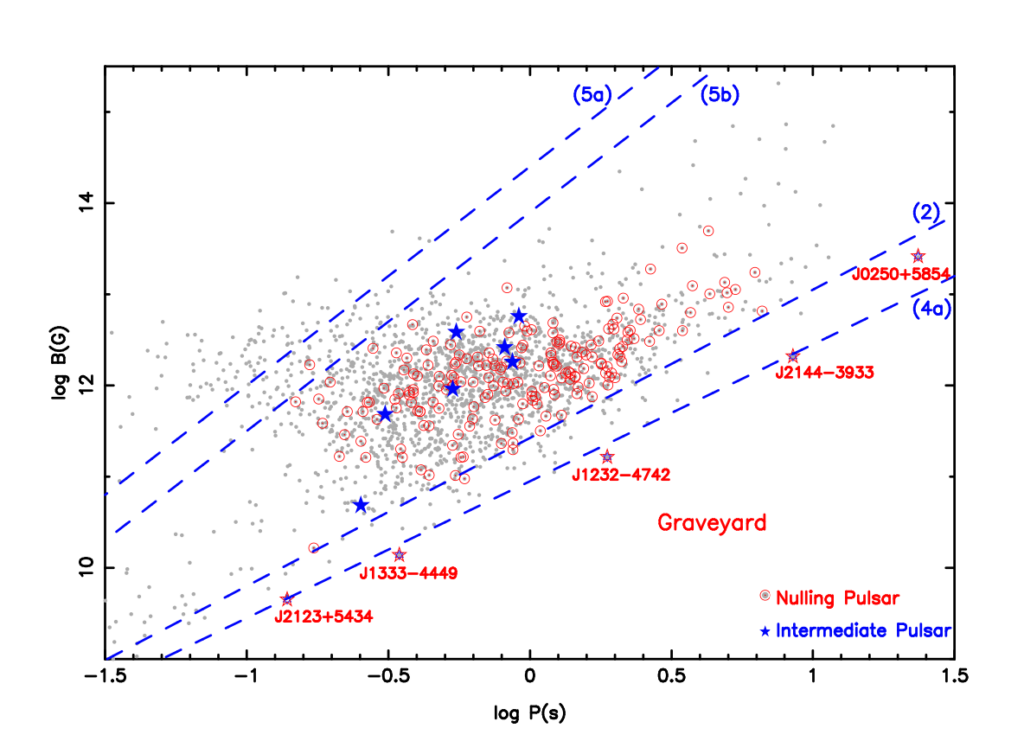
By using a large sample of nulling pulsars, the authors have been able to show a wide gap in nulling fraction and also get closer to pinning down some of the characteristics of nulling pulsars. However, there are still many mysteries to solve. Why are some pulsars beyond the death lines? Why have we not seen nulling in any millisecond pulsars? Only time (and more data) will tell!