Authors: Abhijeet Anand, Dylan Nelson, Guinevere Kauffmann
First Author’s Institution: Max-Planck-Institut für Astrophysik, Karl-Schwarzschild-Str. 1, 85741 Garching, Germany
Status: Accepted to MNRAS.
The circumgalactic medium—the halo of gas surrounding galaxies—could play a large role in galaxy evolution, as it regulates the availability of gas for the galaxy. The origin of this gas is still not completely understood. It can come from the galaxy itself in the form of outflows from active galactic nuclei (AGN) or supernovae, as well as infalling gas from the intergalactic medium (IGM).
One way to study the CGM is using the spectra of bright background sources such as quasars (check out this astrobite for a quick guide on how spectroscopy works!). Quasar spectra (example in Figure 1) show continuum emission that comes from the hot accretion disk, as well as broad emission lines from gas orbiting near the supermassive black hole. Quasar spectra also exhibit absorption lines. Some of this absorption comes from gas in quasar accretion disk winds, but some of it has nothing to do with the quasar at all!
As light from the quasar travels towards us, some of it gets absorbed by the cool gas surrounding galaxies in our line of sight, showing up as absorption lines in the quasar spectrum. Since the wavelengths and shape of these lines are dependent on atomic physics, this tells us what types of elements are present in the CGM and properties of the gas. Many of these studies focus on absorption from singly ionized magnesium (Mg II) gas since it can be observed from the ground and it tells us about the nature of “metals” around galaxies.
The authors of today’s paper looked for this metal-rich cool gas in the spectra of ~1 million quasars from the Sloan Digital Sky Survey (SDSS). Visually inspecting that many spectra would be quite an undertaking! Instead, the authors developed an automated pipeline to detect absorption lines, resulting in the largest catalog of Mg II “absorbers” so far.
The search for absorbers
Figure 1 below shows an example result of the pipeline. To be able to identify absorption lines, the authors first model the continuum emission from the quasar and calculate the residual spectrum (the ratio of the flux data and the continuum model in this case). Any dips in the residual spectrum (bottom panel of Figure 1) below a flux level of 1 indicate a possible absorption line. The pipeline then determines a window of wavelengths to conduct the search, taking care in avoiding any emission and absorption lines from the quasar itself. Once a possible absorption line (a dip, or multiple dips) has been found within the search window, the pipeline compares it to a gaussian model based on the typical line-profile of Mg II atomic transitions. Any possible absorption lines that meet the selection criteria (such as signal-to-noise ratio) are then added to the catalog for further analysis.
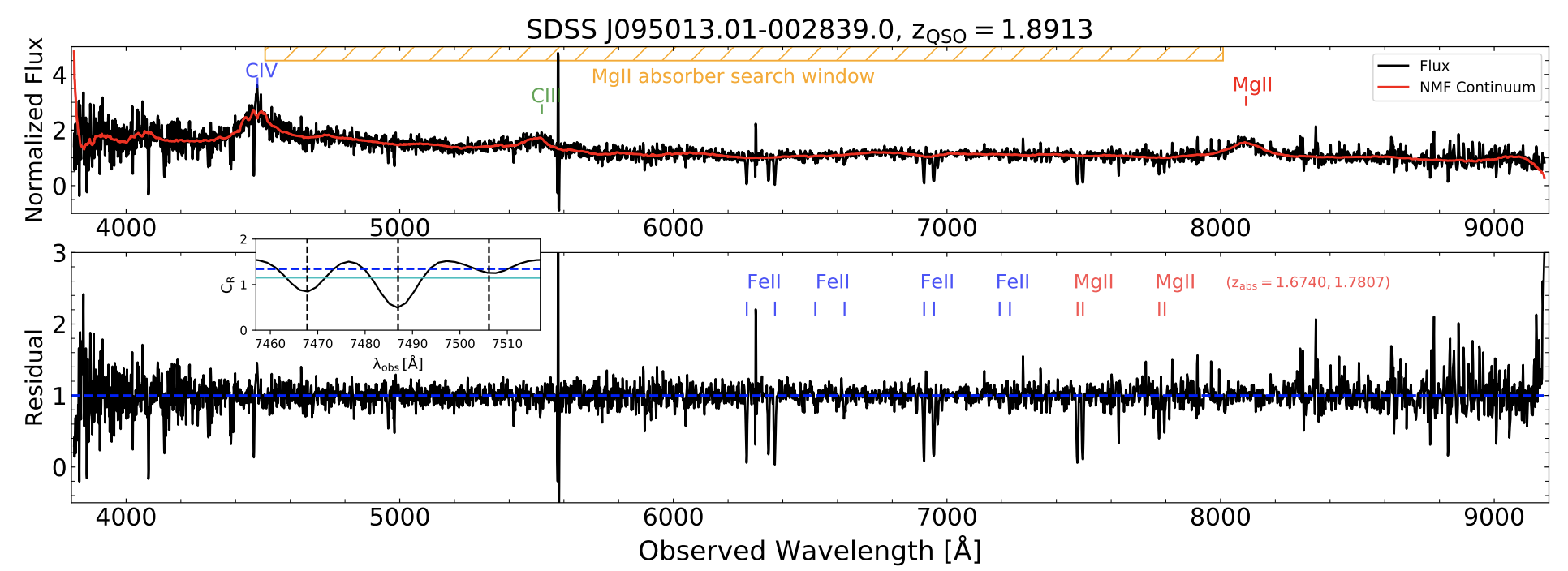
Figure 1. Example result of the pipeline to search for Mg II gas. Top: SDSS quasar spectrum showing emission lines (two carbon lines and Mg II) and a model for the continuum emission (red line). Bottom: Residuals between the normalized quasar flux and the red continuum line. Possible absorption lines from Mg II and iron (Fe II) are shown. (Figure 1 in the paper).
In total, the authors find a sample of ~160,000 Mg II absorbers and analyze their properties such as equivalent width, as well as try to identify any additional, less prominent absorption from other metal gases like silicon and carbon which are also usually present in these absorbers.
Galaxy properties
In order to understand the relationship between the gas that surrounds galaxies and the galaxies themselves, the authors look at two subsets of galaxies: emission line galaxies (ELGs, star-forming) and luminous red galaxies (LRGs, no star formation). These galaxies were also obtained from the SDSS.
For each of these galaxies, the authors calculate the covering fraction of Mg II gas, that is, the ratio of the number of Mg II absorbers around a galaxy, divided by the number of quasars that are visible through that galaxy. This helps them understand how common these absorbers are for each kind of galaxy. The authors find a clear difference between covering fraction for ELGs and LRGs, with ELGs having a covering fraction a factor of 2-5 times higher than LRGs at small distances.
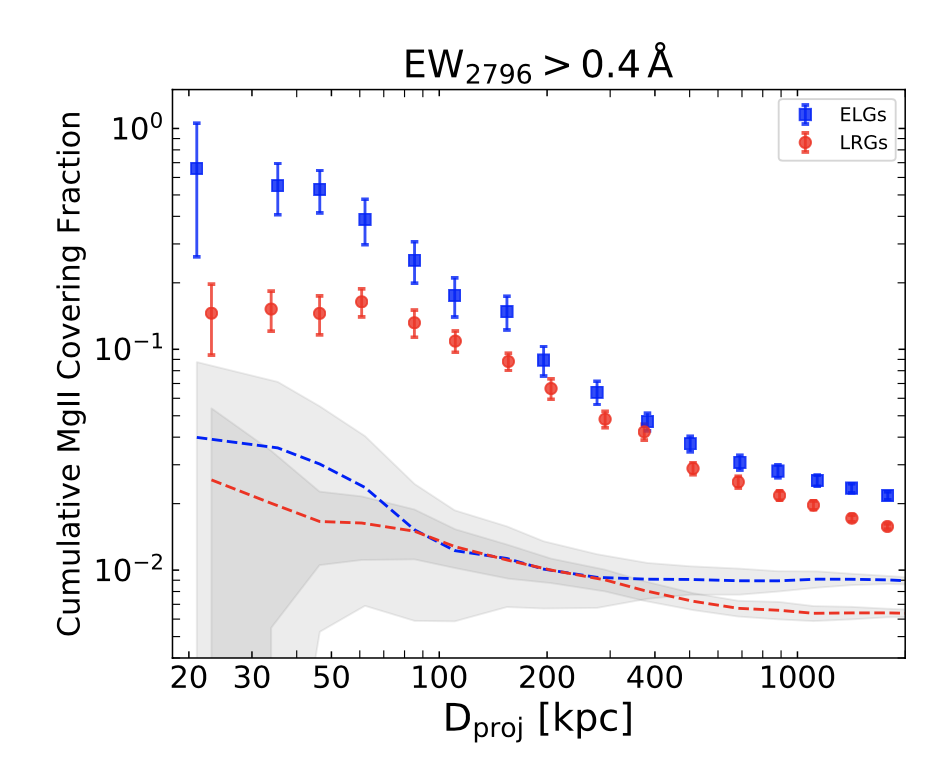
Figure 2. Covering fraction (defined in the text above), as a function of projected distance from the galaxy. The blue points show the results for emission line galaxies, and the red points show the results for luminous red galaxies. (Figure 13 in the paper).
The authors also look at the dependence of this covering fraction with galaxy properties like the stellar mass and star formation rate (SFR). For the LRGs, there is an anti-correlation between the covering fraction and the mass of the galaxy that comes from stars. The more massive the LRG, the less cool gas it has surrounding it.
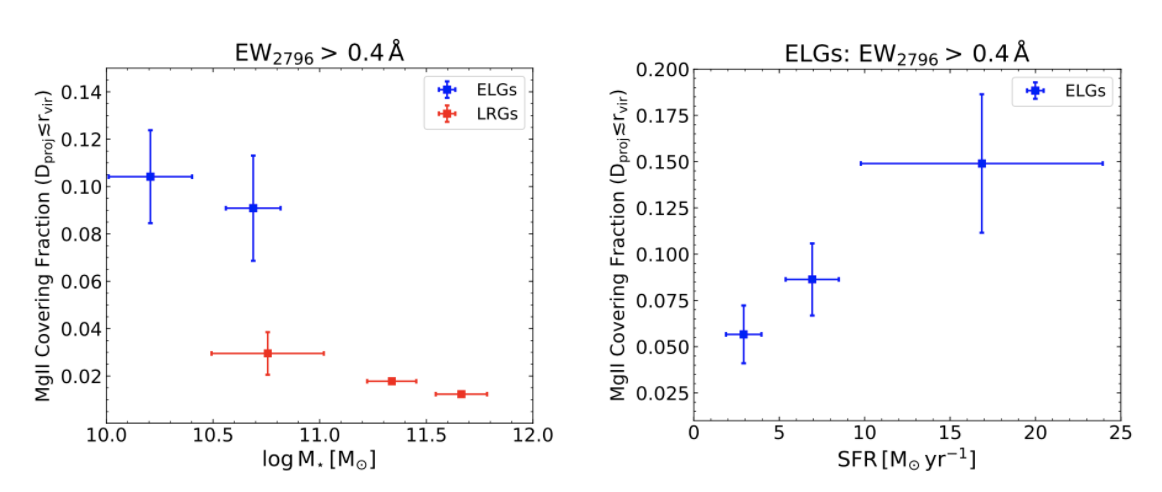
Figure 3. Left: Covering fraction vs stellar mass for ELGs and LRGs. (Top panel of Figure 15 in the paper). Right: Covering fraction vs star formation rate for ELGs. (Bottom panel of Figure 17 in the paper).
For ELGs, the trend with stellar mass is not so clear, however, they do show a strong correlation between covering fraction and star formation rate. ELGs with high star formation rates have a much higher Mg II absorber covering fraction than those with lower star formation rates.
These different trends for ELGs and LRGs of covering fraction with galaxy properties could hint at a different physical origin for these Mg II absorbers that depends on whether the galaxy is star-forming or not. The CGM around a star-forming galaxy for example, might have more gas that comes from outflows. Large systematic searches like these set the stage for future large scale surveys to better understand the nature of cool metal-rich gas surrounding galaxies.
Astrobite edited by Katya Gozman
Featured image credit: ESO/M. Kornmesser