Title: Optical Appearance of Eccentric Tidal Disruption Events
Authors: Fangyi (Fitz) Hu, Daniel J. Price, and Ilya Mandel
First Author’s Institution: Monash University, Australia
Status: Submitted to the Astrophysical Journal Letters [open access]
While the typical image of a supermassive black hole swallowing up everything in its path may be a trope of science fiction, there is some truth to the violent nature of these enigmatic cosmological objects. Namely, the ultra-strong gravitational fields surrounding supermassive black holes have been observed to shred stars, tearing them apart and producing bright and dramatic transient phenomena known as tidal disruption events, or TDEs. In the process, much of the stellar material becomes unbound and is flung away from the system, with the remainder (less than 10%) forming an accretion disk around the star, the source of the emitted radiation.
As per usual though, nothing in astrophysics is ever straightforward. Over the years, a disparity between what we expect and what we actually see has emerged when it comes to these events reminiscent of a Shakespearean tragedy. More specifically, due to the very high-temperature gases involved, X-rays are expected to dominate the spectrum of most TDEs. However, observers have found that most TDEs are instead observed at lower energies, producing significant but poorly understood optical emission.
Enter today’s paper from researchers at Monash University in Australia, who have simulated the tidal disruption of a sun-like star in the hopes of uncovering the origin of the perplexing optical emission.
The Nitty Gritty of the Simulation
For simulations to be realistic, theorists typically attempt to account for as many physical processes as possible. Thus, the authors of today’s paper employed the PHANTOM code, which includes both general relativistic and hydrodynamical effects. The protagonists of this astrophysical tragedy are a solar-mass star and a million solar-mass, non-spinning black hole with a highly eccentric, or elliptical, orbit.
Cut to several days later, and the simulation has evolved to a point where the star is in tatters, and the supermassive black hole is surrounded by a bright and brand-new accretion disk composed of stolen material from the star. By tracking the time evolution of the system, the authors were able to understand how the accretion disk formed, with the hope of unmasking the source of the optical emission.
The Last Dance
Figure 1 shows the column density evolution of the system, indicating the location of the stolen stellar material. In the first two panels at 0.55 and 0.91 days, the stellar debris stream is visible. In the third panel, the debris stream collides with itself, leading to the formation of the accretion disk. In the fourth panel at 2.74 days, the accretion disk is clearly seen as a circular structure surrounding the supermassive black hole at the centre.
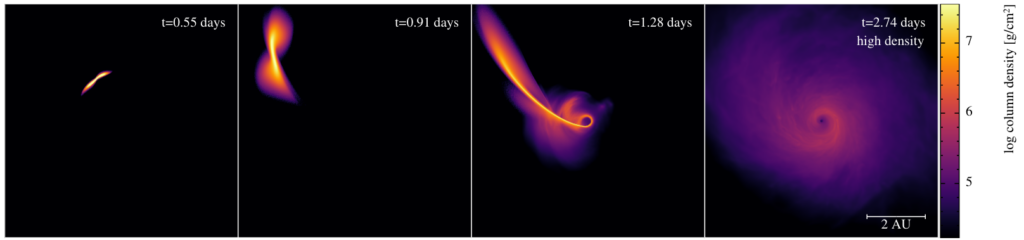
However, by considering the system from afar, the authors gained a new perspective on TDE accretion disk formation. They found significant quantities of low-density material ejected out to large distances. This material is shown in Figure 2, which gives the column density of the system at 2.74 days as in Figure 1, but on a much larger scale.
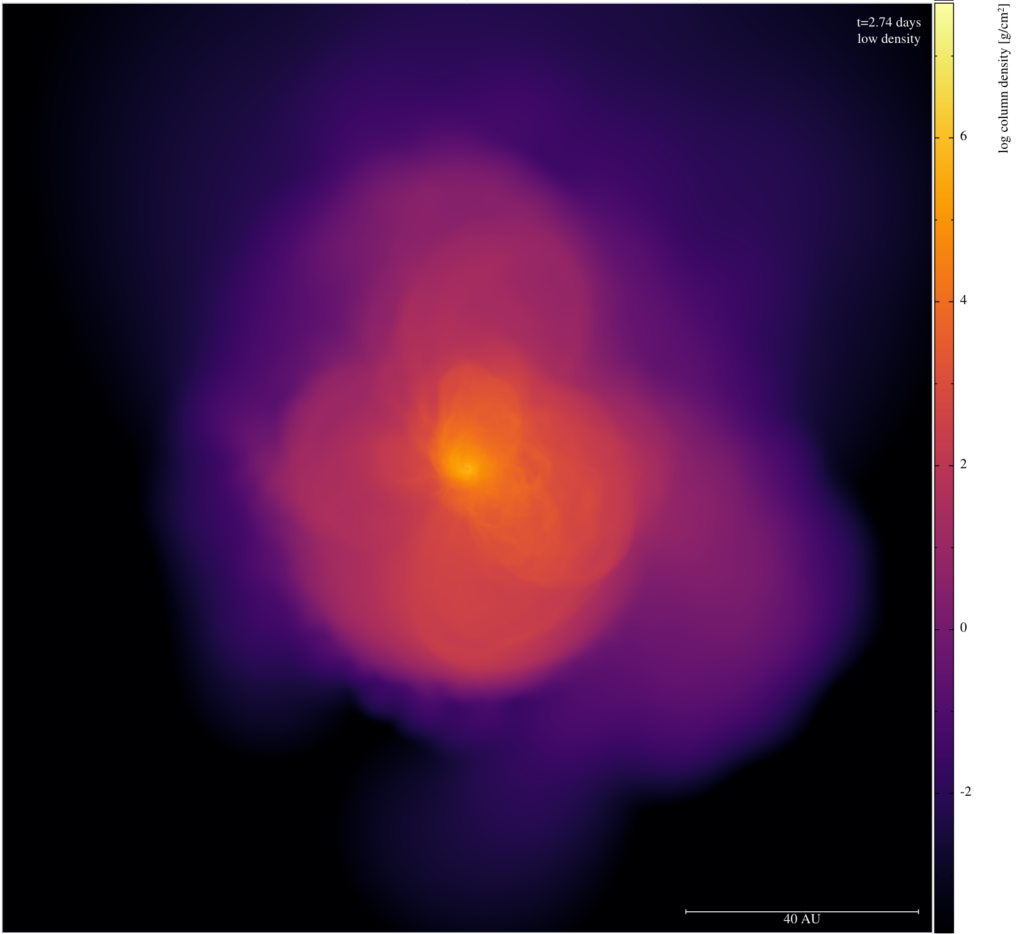
This low-density material is not part of the accretion disk but instead flows out asymmetrically from the centre of the system. Previous studies have hypothesised that the source of the optical emission from TDEs might be some form of reprocessing layer that surrounds the accretion disk and converts X-ray photons into lower-energy optical photons. And that appears to be exactly what is seen in the simulation. The surrounding low-density material in Figure 2 acts as the reprocessing layer with which X-ray photons interact, causing them to lose energy and be observed as optical emission.
But how does this match with actual TDE observations? To test this, the authors constructed spectra and light curves from their simulation results. They found that their synthetic spectra were broadly consistent with the genuine TDE spectra, as were their inferred luminosities from their synthetic light curves. However, their calculated blackbody radii and temperatures were systematically higher or lower than the observations, albeit in the correct order of magnitude. Therefore, the authors conclude that while the model is realistic, it does not yet accurately account for certain physical processes such as radiation transport, which can be improved upon in future simulations.
And thus ends the tragic tale of a supermassive black hole and its stellar neighbour that came a little too close. Clearly, surrounding outflows play an important role in this astrophysical tragedy that rivals Hamlet or Macbeth, processing the high-energy X-rays from the accretion disk into the lower-energy optical photons seen by observers. Unfortunately for us though, we’ll have to wait for future simulations to find out how the story truly ends.
Astrobite edited by Archana Aravindan
Featured image credit: NASA/JPL-Caltech