• Title: Constraints on off-axis jets from stellar tidal disruption flares
• Authors: Sjoert van Velzen, Dale A. Frail, Elmar Koerding, Heino Falcke
• First Author’s Institute: IMAPP, Radboud University, the Netherlands
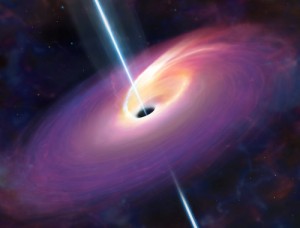
An artist’s impression of the formation of jets as a result of a tidal disruption event. Image credit: University of Warwick / Mark A. Garlick.
We’ve had quite a bit to say in the past about the phenomenon of tidal disruption events (TDEs). These events occur typically when an object gets too close to a black hole and consequently gets torn apart by the strong tidal forces that the black hole exerts. The shredded object — which might have been an asteroid wandering too close to a stellar-mass black hole, or a star that didn’t give a super-massive black hole wide enough berth — then accretes onto the black hole, releasing a sudden burst of energy known as a tidal disruption flare (TDF).
The thing is, this kind of high rate of accretion is almost always associated with the production of relativistic jets. These jets can be seen in things like active galactic nuclei, microquasars, and gamma-ray bursts, all in the context of rapid accretion onto a black hole. So this raises a very reasonable question: could all TDEs also generate relativistic jets?
The authors of today’s paper hypothesize “yes” — and then set about trying to prove it.
The Problem with Previous Observations
Relativistic jets are generally identified by their radio signature (which is due to synchrotron emission along the entire length of the jet), so testing for them requires making radio observations of TDEs to determine if the flare is accompanied by a radio jet. Tens of candidate TDEs have been observed so far, falling into two categories: there are gamma-ray observations made by Swift of two events, and the remaining events were imaged in soft X-ray or optical wavelengths and are termed “thermal” flares. Observations of all TDFs so far have only detected a radio counterpart for the two gamma-ray-detected events. Of the remainder, some haven’t been followed up in the radio wavelength, but those that have show no radio emission: they are radio-silent.
Testing the Hypothesis
The authors of today’s paper suggest that all TDEs result in relativistic jets, and they argue that the primary explanation for the radio-silence of the thermal TDFs is simply that the jets aren’t pointing toward us. Relativistic emission gets beamed in the direction of motion, boosting its apparent luminosity only along that one direction and dimming the brightness in all other directions. That means that if the jet axis isn’t near the line of sight to Earth (i.e., if we’re off-axis), we might miss it entirely!
The work in this paper attempts to address this possibility in three steps:
1) The authors perform radio follow-ups of additional optical/UV TDFs that all occurred 1–8 years ago. Next,
2). They build models of what we might expect the light curves to look like from off-axis, assuming these TDEs had jets. And finally,
3). They test their hypothesis that all TDEs have jets by calculating the probability that the observations are consistent with the jet models.
The authors test three models: an optimistic scenario ((a) in the figure below) where the jet is radio-loud always, a conservative scenario (b) where the jet is only radio-loud after a time when the accretion rate has dropped below a certain value, and an in-between scenario (c) where the jet has an initial radio-loud burst during the onset of accretion and then quiets down. Model (c) is considered to be the most realistic scenario.

Probability of the data being consistent with three models (denoted by the three different colors) of TDEs with jets. The solid lines show the probability that all seven observations hosted a jet; the dashed lines show the probability that at least one hosted a jet.
Results
The authors obtained radio observations with the Jansky VLA of seven new TDEs. When compared with the models they created, the data end up having a fairly low probability of being consistent with the “all TDEs have jets” hypothesis.
The probability is dependent upon the speed of the jet — described in relativistic physics by the jet’s Lorentz factor — since the jet’s speed determines to what extent the radiation is beamed away from an off-axis observer. The figure to the right shows that for a Lorentz factor under 3, the possibility of all seven TDEs hosting a jet can be ruled out with 95% confidence under all three of the models.
The results are less confident for higher Lorentz factors, but if this does indeed suggest that not all TDEs produce jets, then the obvious next question to ask is: what determines whether or not a jet is produced in a TDE? A common theory is that the jet-launching mechanism is sensitive to properties of the system, such as the black hole spin, or the environment around the black hole.
The authors conclude by suggesting that future radio transient surveys such as VAST, ThunderKAT, or LOFAR will provide the next step toward settling this question: either they’ll detect tens to hundreds of tidal disruption jets per year, or else they’ll discover that the rate of TDEs with jets is much lower than the rate of radio-quiet TDEs detected.