Title: Detection of extended gamma-ray emission around the Geminga pulsar with H.E.S.S
Authors: H.E.S.S. Collaboration
Status: Accepted by A&A, open access
Pulsars, nebulae, and pulsar wind nebulae, oh my!
Pulsars are understood to be the rapidly spinning cores of massive stars after they’ve exploded into supernovae at the end of their lives. They’re often brightest at radio wavelengths, which is usually the first (and often only) wavelength pulsars are seen to pulse in.
At very high energy (VHE; > 100 GeV) gamma-ray wavelengths, a nebula surrounding a pulsar, called a pulsar wind nebula (PWN or PWNe in plural), is of particular interest. This nebula is found inside the shell of the supernova remnant nebula (see Figure 1), and is formed as winds from the central pulsar expand into their surroundings. It’s powered by non-thermal, particle physics processes (rather than thermal processes like absorption, etc.), which are brightest in radio wavelengths, X-rays, and gamma-rays.
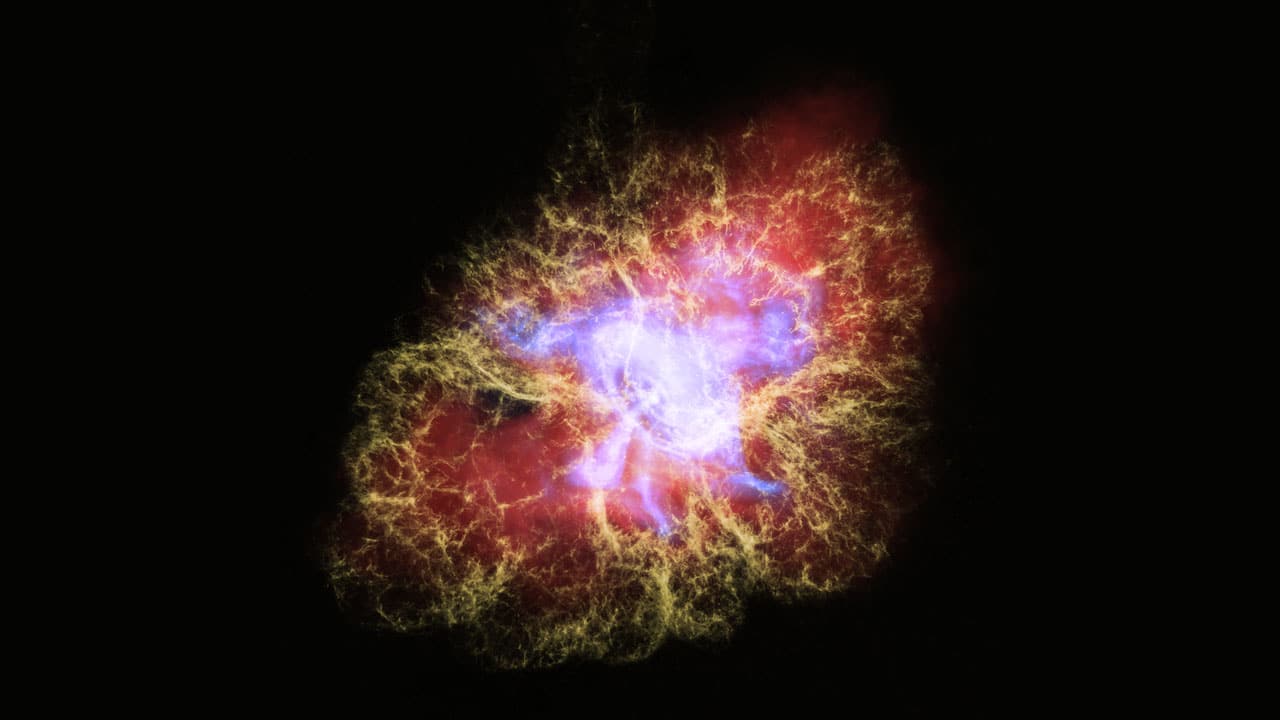
Tracking down the highest energy particles in the universe
Two of the biggest questions in VHE astrophysics at the moment are thought to be answerable by further studies of PWNe. One of these questions is to figure out what is powering PeVatrons (cosmic accelerator systems capable of accelerating particles to PeV energies; i.e., over 100x the energy of the LHC!) and whether or not PeVatrons are producing charged cosmic rays (mostly protons) AND photons, or just cosmic rays. This is particularly interesting, because while most cosmic rays are diverted in their paths by magnetic fields, uncharged photons can easily be traced back to their birthplaces, making it much easier to figure out what’s emitting them (if we can model emission with something that produces both cosmic rays and photons). One of the leading theories is that PWN are capable of being PeVatrons, but there are very few PWNe that are easily seen and studied by today’s generation of ground based gamma-ray telescopes (called imaging atmospheric Cherenkov telescopes or IACTs), making it difficult to constrain whether or not this is the case.
There are only a few PWNe that are close enough to Earth that we can observe them as extended. Extended emission is basically just anything larger than the telescope’s point spread function, meaning that we can see (and therefore study!) some structure and morphology, rather than just a dot. The Crab pulsar and PWN are very young (which usually means very energetic) and close to Earth, making it a very easy PWN to study. However, in order to better understand PWN evolution and emission, we need to study older systems as well. The Geminga pulsar is a particularly intriguing example of an old PWN, as it is also very close and its PWN is much larger than that of the Crab and any other that has been studied at TeV energies. IACTs have been trying to detect Geminga at TeV energies since the mid-2000s, but it has long eluded detection. Until today!
Detecting the (previously) undetectable
Today’s authors report the first IACT detection of Geminga using the High Energy Stereoscopic System (H.E.S.S.), an array of five IACTs located in Namibia. Though H.E.S.S. has been observing Geminga since 2006, it has taken a long time to tune observations and analysis to capture the full region accurately. The full gamma-ray emitting region of Geminga is huge (> 2 degrees; or at least 4 times the angular diameter of the Moon in the sky!), which fills up most of H.E.S.S.’s camera. Because of the significant background of gamma-rays and cosmic rays in the sky, IACTs need to be able to get a background region in their cameras in order to determine if the source they’re looking at has an excess of gamma-rays over the background.
IACTs use a neat trick called ‘wobble’ to increase their background regions with limited field of views of typically 3-5 degrees. Typically, IACTs point their cameras slightly offset from the source in order to capture a four-leaf clover shaped region with the source of interest at the centre. This wobble is usually only about 0.5 degrees, but after many years of not seeing Geminga, H.E.S.S moved to a wobble of 1.6 degrees – one of the biggest offsets ever used by an IACT!
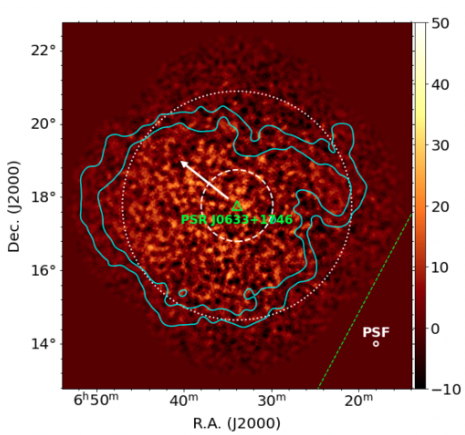
Big nebula, even bigger questions!
So why is Geminga’s emission region so huge? Extended gamma-ray emission is usually a good way to figure out how particles are transported within a region. In pulsar environments, particles are accelerated to high energies near the pulsar’s jet, and then move around the surrounding nebula, interacting with their environment to produce gamma-rays as they go.
Even after successful detection of Geminga’s PWN, the authors made an interesting discovery. It turns out that the PWN is even bigger than was previously thought. The authors count the total number of gamma-rays in wider and wider rings moving outward from the central pulsar (see figure 3), which is expected to be constant after getting to a large enough radius, where gamma-rays are no longer being produced. They found that even in the huge field of view they captured, they saw no evidence for this ‘flattening’ (see figure 2). By fitting this curve to known models, it’s expected that gamma-ray emission extends out to 50 pc, which is twice H.E.S.S’s field of view. This means we will need to wait a little longer for next generation of gamma-ray instruments (like the Cherenkov Telescope Array – CTA) to come online before being able to fully capture Geminga’s PWN in VHE energies.
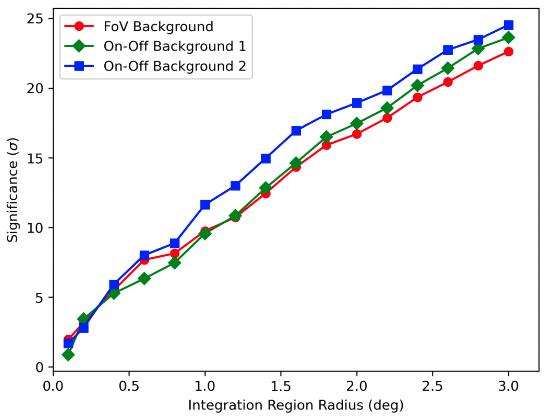
The authors also compared their TeV energy data points to those from Fermi-LAT (GeV gamma-rays) and X-ray instruments to constrain the maximum energy that Geminga’s PWN can emit photons at. It looks like this energy cutoff is around 75 TeV, which disfavours Geminga as a PeVatron candidate (which would need it to be emitting gamma-rays around 1000 TeV). However, since the analysis done by H.E.S.S. was the first of its kind to study these huge gamma-ray emitting regions, there are still pretty big uncertainties on the data. Using newer, larger gamma-ray telescopes like CTA and ultra high energy instruments like LHASSO can help us get more robust constraints on the ability of older PWN to be PeVatrons as well as detecting new PWN will be essential to answering some of today’s big questions about PeVatrons and cosmic rays.
Astrobite edited by Lynnie Saade
Featured image credit: Chandra X-ray Observatory