- Title: Multiple planets or exomoons in Kepler hot Jupiter systems with transit timing variations?
- Authors: R. Szabó, Gy. M. Szabó, G. Dálya
- First author’s institution: Konkoly Observatory, Budapest, Hungary
The Big Picture
Astronomers have noted that hot Jupiters (close-in gas giants) tend to be the only planet orbiting their host stars. The scarcity of other planets in systems with hot Jupiters led astronomers to coin the phrase, “hot Jupiters are lonely.” In this paper, Szabó et al. search for evidence of other planets and exomoons in the hot Jupiter systems in the Kepler data set by inspecting the times at which the hot Jupiter transits its host star. If all hot Jupiters are truly lonely (meaning that the system consists only of the host star and the hot Jupiter), then the transit times should be strictly periodic. However, a massive exomoon orbiting the hot Jupiter or an additional planet orbiting the host star would gravitationally tug on the hot Jupiter and cause the transits to occur periodically early or late. There are several other reasons why the transit times might appear to vary, however, and Szabó et al. find that the observed transit timing variations of most (but not all!) of the hot Jupiters in their sample can be explained by the timing of the Kepler observations and the variability of their host stars.
Excitingly, sixteen of the hot Jupiters in their final sample exhibit transit timing variations that cannot be attributed to systematics. By assuming that the transit timing variations are not caused by some unknown mechanism, then one would expect that these hot Jupiters must either host exomoons or reside in systems with at least one other planet. The authors argue that the additional planet hypothesis is more likely than the exomoon hypothesis because exomoons orbiting hot Jupiters are unlikely to remain stable given the strong tidal forces between the exomoon, hot Jupiter, and host star. Additional observations of these fascinating systems will help unravel the mystery of whether hot Jupiters really are lonely.
The Data
The most recent list of Kepler planet candidates contains 159 hot Jupiters with at least 12 transits and reliable orbital periods. Szabó et al. began with that list of hot Jupiters and obtained the transit times for each planet from the catalog published by Ford et al. 2012. The authors then took the Fourier transform of the data and looked for peaks in the frequency distribution of the transit time variations. Fourier transforms for six systems are shown in the figure below. Peaks found in the Fourier transforms are evidence that the transit timing variations are being periodically perturbed, but there are several different explanations for why the transit times could vary.
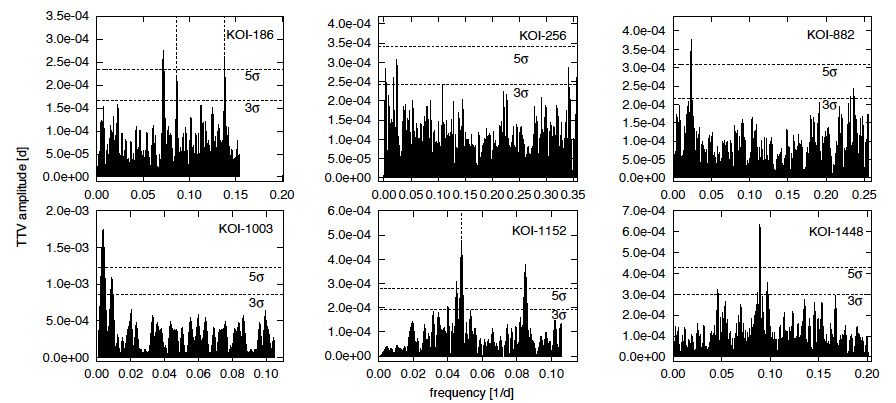
Transit timing variation amplitude versus frequency for six different hot Jupiters. The horizontal lines indicate the 3-sigma and 5-sigma detection thresholds and the vertical dashed lines indicate transit timing variations that are due to systematics. The transit timing variations not marked by vertical dashed lines could be due to unknown effects or perturbations from other planets or exomoons. Figure 3 from Szabo et al. 2012.
Why Do Transit Times Vary?
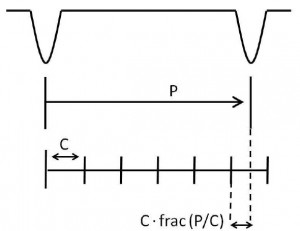
Schematic showing why regular sampling of the light curves induces a "virtual" transit timing variation. The line at the top shows two consecutive planet transits separated by the orbital period P. The bottom line marks the sampling rate C. The brightness of the star is measured at the center of the first transit, but the measurements are offset by C times the fractional part of P/C during the second transit. The systematic lag in the sampling times produces a "virtual" transit timing variation. Figure 2 from Szabo et al. 2012.
The most interesting explanation for transit timing variations is that the planets are being gravitationally perturbed by other planets in the system or by their own exomoons. Alternatively, transit timing variations can also be induced by the the sampling rate used when collecting data. Most Kepler observations are taken in long cadence mode, meaning that the brightness of the star is measured once every 29.424 minutes. For a typical hot Jupiter, this means that only 3-6 brightness measurements are acquired during each transit and that those measurements are obtained exactly 29.424 minutes apart. The placement of the measurement points within each transit will shift slightly over time because the 29.424 minute sampling rate does not divide evenly into the planet’s orbital period, but the regular temporal spacing of the measurements can introduce a periodic variation in the transit times as depicted in the sketch at right. The expected frequency of the “virtual” transit timing variation due to the sampling frequency can be calculated from the sampling frequency and the planet’s orbital period, so Szabó et al. are able to tell which transit timing variations are due to the sampling rate.
Some transit timing variations can also be attributed to the magnetic activity of the host star. If a host star has starspots, then the light curve will show brightening and dimming events as starspots form, disappear, and rotate into view. The timing of the brightening and dimming events is governed by the rotation period of the star and the starspot lifetime, so the periodicity of the events can produce transit timing variations. Szabó et al. exclude a few systems from their analysis because the periods of the transit timing variations are correlated with the stellar rotation period.
Conclusions
After carefully inspecting their sample, Szabó et al. eliminate all but 16 of the 29 hot Jupiters with transit timing variations. Assuming that all of the systematics have been considered, these hot Jupiters must be perturbed by a third body. Exomoons are unlikely to form and survive around hot Jupiters, so Szabó et al. believe that the most likely explanation is that the 16 hot Jupiters reside in systems with additional planets. If this theory is correct and these hot Jupiters aren’t lonely at all, then astronomers will need to revise our theories about hot Jupiters to figure out why some hot Jupiters are lonely and others reside in multiplanet systems. Szabó et al. point out that longer-duration and higher-precision observations are required to confirm the presence of additional planets in hot Jupiter systems, so stay tuned for future astrobites about the loneliness of hot Jupiters.