Title: Water Trapping on Tidally Locked Terrestrial Exoplanets Requires Special Conditions
Authors: Jun Yang, Yonggang Liu, Yongyun Hu, and Dorian S. Abbot
First Author’s Institution: University of Chicago
Status: Accepted to The Astrophysical Journal Letters
We’ve talked many times before on Astrobites about habitable exoplanets, defined by whether they can retain liquid water on their surfaces. To first order, this involves the habitable zone, otherwise known as the “Goldilocks zone” or the “just right” distance a planet can orbit its star where water on the planet’s surface will neither freeze solid nor be boiled away. But distance from the star (which translates directly into the amount of stellar radiation received by the planet) is only the first-order approximation; to truly understand the state of a planet’s water, we have to understand the details of the planet’s atmosphere and heat circulation. This is mostly beyond today’s observational abilities, but we can apply models to tell us what kinds of planets are good candidates for habitability.
Yang and collaborators explore a specific subset of exoplanets: tidally locked, rocky exoplanets orbiting M-stars. M-stars are the most common type of star by far, and because they are small, cool stars, their habitable zones are located close in. This results in tidal locking for many habitable zone planets. Tidally locked planets orbit such that one side always faces their star, and one side out into space. Consequently, they have huge temperature gradients between their day and night sides. Atmospheric circulation in these planets will tend to transport water from the day side of the planet (where it evaporates) to the night side (where it condenses back out of the atmosphere). Once on the cold night side, it will freeze and potentially be trapped as ice. But how much of this water freezes? Once it freezes, is it lost forever as a liquid? To understand this scenario more fully, atmospheric circulation models must be combined with models describing the flow of oceans as well as both land and sea ice sheets.
Yang and collaborators use models developed to study Earth’s climate, which comprise coupled models to study atmosphere, ocean, sea ice, and land. In all cases they examine a typical super-Earth exoplanet with a period of 37 days, a radius of 1.5 Earth radii, and a gravity 1.38 times that of Earth, but with a variety of continent/ocean configurations. They examine a waterworld with no continents and three different ocean depths, a planet with one supercontinent covering the night side and an ocean on the day side, with uniform elevation and depth, respectively, and a planet that looks like modern-day Earth, with a substellar point in either the Atlantic or Pacific Oceans, or in Africa.
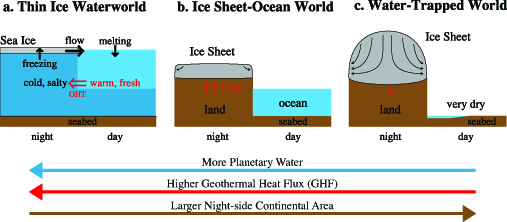
Figure 1. Diagram showing amount of water for three different types of planets. On the left is a waterworld, where water and ice are transported easily between the hot day and cold night sides, resulting in little ice trapping. In the center, a planet with a large continent covering the night side still maintains a sizeable ocean, because the high heat flux of the planet keeps the ice sheet small. In the right panel, also with a night side continent but with a lower heat flux, the continental ice sheet grows, trapping most of the water of the planet. This last is the worst case scenario for habitability, but is only possible when the heat flux is low, the continents are all on the night side, and the overall water reservoirs are small compared to Earth’s water stores. (Credit: Yang et al. 2014)
For the waterworld, they find that the ice on the night side becomes only 5.4 meters thick, leaving plenty of liquid water on the planet. While ice forms on the night side, it is also continuously melted by warm ocean currents circulating from the day side, and by surface winds that push the ice sheets towards the warmer substellar point. Yang adds continental barriers here, running north to south on the eastern and western terminators (the day/night dividing lines, a fixed geographical point on a tidally locked planet), to investigate what happens if the ocean and ice transport is disrupted by a land barrier. In this case, the ice grows to 1000 meters thick, effectively trapping the water as ice.
On a planet with one supercontinent on the planet’s night side, the water that is trapped as continental ice sheets is maximized for a low geothermal heat flux. For a planet with Earth’s water stores and heat flux, roughly half its ocean would be trapped in such a scenario. For a super-Earth, which would likely have a higher heat flux, only a small ocean, a few hundred meters thick, would be trapped. See Figure 1 for a comparison between this scenario and the waterworld.

Figure 2. For a planet with modern-day Earth’s continental configuration, ice sheet thicknesses are shown for the oceans (left) and land (right). The color bar shows the ice thickness, contours show surface air temperatures of 0, 5, and 7 degrees C, and arrows indicate sea-ice velocity. The black dot indicates the substellar point. This is for a planet with a low heat flux. Most tidally locked super Earths would probably have a higher heat flux, resulting in much thinner ice sheets. (Credit: Yang et al. 2014)
If less artificial continents are studied, such as modern-day Earth’s continental configuration, the ice remains ~10 meters thick in most areas, though it can grown to ~100 meters in a few isolated regions like Baffin Bay or mostly inland seas. Even small passages between continents allow enough transport of sea ice and water currents to avoid trapping a critical amount of water in ice. See Figure 2 for details.
In conclusion, the habitability outlook for these tidally locked planets is pretty good! Ocean planets can efficiently transport ice back to the day side to be melted, and even small breaks in continental coverage are enough to prevent critical amounts of water being trapped in ocean or land ice sheets. It will be difficult to detect the differences between these kinds of planets observationally, but looking at reflectivity measurements could indicate land/water/ice coverage on planets.
Wow this is so exciting! Do most M Stars have terrestrial planets around them or do many have more gaseous planets? Are these habitable planets a very common occurrence?