Title: How much water was delivered from the asteroid belt to the Earth after its formation?
Authors: Rebecca G. Martin, Mario Livio
First Author’s Institution: University of Nevada
Status: Accepted for Publication in MNRAS Letters, available on arXiv
Water is often considered Earth’s most precious resource, and its vast liquid oceans make Earth unique among the terrestrial planets in the solar system. Despite this, the origins of Earth’s H2O remain something of a mystery, with theories of how terrestrial planets obtain water varying from delivery by comet and asteroid impacts, to generation through atmosphere-magma interactions.
Some of this confusion comes from the fact that the precise amount of H2O in and on the Earth isn’t certain. Earth is thought to have somewhere between 1 and 10 “oceans” worth of water, where 1 ocean is equal to the amount of water on the surface, meaning that there could be several oceans worth of H2O within the Earth’s mantle. Different theories as to the origins of water on Earth provide different quantities of H2O – for example, the meteorites that helped to form our planet could have provided as many as three oceans worth of water. Today’s paper concerns just how much H2O might have been delivered to Earth from the asteroid belt, as its authors investigate asteroid collisions with Earth once it had formed.
How Often Do Asteroids Hit Earth?
To estimate how much water could have been delivered from the asteroid belt, the authors first simulated the orbital evolution and fate of asteroids in different regions of the belt over 10 million years, to determine how many collisions the Earth might experience. In the model, asteroids could have one of several outcomes, either remaining in the asteroid belt, leaving the belt and being ejected into space, or leaving the belt and colliding with a planet or the sun. During each simulation, the authors placed 10,000 asteroids in one of three narrow regions of the belt – a resonance region at 2.1 AU known as the ν6 resonance, the 2:1 mean motion resonance region with Jupiter at 3.3 AU, and a zone on the outer edge of the belt at 4 AU. The fates of the asteroids in each simulation were then used to estimate the probability that an asteroid leaving each region would collide with Earth.
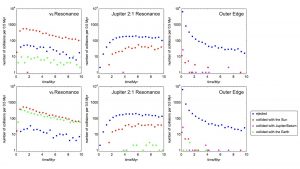
Figure 1: Asteroid outcomes for each region of the asteroid belt over the 10 million year simulation. For the asteroids which left the belt, each panel shows how many asteroids were ejected into space (blue points), collided with the sun (red points), collided with Earth (green points) or collided with another planet (pink points). The top row shows the simulation results for an Earth-sized Earth for each asteroid belt zone, while the bottom row shows the results when Earth is replaced by a planet 10 times the size of Earth. Adapted from Figure 1 in the paper.
As shown by the green points in the top panels of Figure 1, only the ν6 resonance region resulted in asteroid-Earth collisions, with 113 in total, giving an asteroid-Earth collision probability of 1.9%. In order to obtain probabilities for the regions that saw no collisions, the simulation was repeated with a planet ten times the radius of Earth, as a larger planet should experience more collisions, as seen in the lower panels of Figure 1. The resulting asteroid-planet collision probabilities were then scaled back to account for the larger cross-section of this planet compared to Earth, providing an estimate for the collision probability for the real Earth. Using this method, the 2:1 Jupiter mean motion resonance region was found to have an asteroid-Earth collision probability of 0.02%, while the probability for the outer edge of the asteroid belt was just 0.0025%, making both regions unfavourable for providing Earth with water.
Do Asteroids Contain Enough Water?
While the simulations make it clear that the inner ν6 resonance region is the most efficient at delivering asteroids to Earth, it is the asteroids in the outer regions of the belt that contain the most water, so how much water could the ν6 zone actually deliver? Asteroids in the outer regions of the belt can move further inwards through interactions with other asteroids, or mechanisms such as the Yarkovsky effect, in which asteroids change momentum, and hence their orbits, as they radiate the heat imparted on them by the sun. This means that the more water-rich asteroids in the outer regions could be moved into the ν6 resonance zone, increasing their Earth collision probabilities.
Through this mechanism, the authors estimate that asteroids leaving the ν6 resonance could provide Earth with as many as 8 oceans worth of H2O. Although this would easily supply enough water for the Earth’s surface, it would not account for the upper limit of water in and on the Earth, equal to 10 oceans. The authors therefore conclude that if the Earth’s mantle contains large reserves of water, the Earth must have formed with significant amounts of it, once again establishing that without a firm measurement of the H2O content of Earth, the origins of Earth’s water will remain uncertain.
Astrobite edited by Lukas Zalesky
Featured image credit: Simone Marchi / SwRI
This blog post is a captivating exploration of a topic I hadn’t considered before- the contribution of the asteroid belt to Earth’s water reserves. It is a fascinating reminder of how interconnected bodies and how these cosmic interactions shape our planet.
Kudos to the author for shedding light on this celestial water transfer, expanding our understanding of Earth’s history.