Title: The post-transit tail of WASP-107b observed at 10830Å
Authors: J. J. Spake, A. Oklopčić, L. A. Hillenbrand
First Author’s Institution: Division of Geological and Planetary Sciences, California Institute of Technology, Pasadena, CA 91125, USA
Status: Submitted to AJ [closed access]
Since the ground-breaking confirmation of the first exoplanet in 1992, astronomers have been finding and characterizing thousands of alien worlds in hopes of understanding the mechanisms behind planetary formation and evolution. Unsurprisingly, we get very excited about finding planets similar to ours, located in the “Goldilocks Zone” where potential life could thrive. But let’s not neglect the hidden gems that orbit much closer to their host star: not only are these among the first exoplanets we ever found, but their close proximity to their host stars gives us the unique opportunity to detect something that we wouldn’t be able to see otherwise: their atmospheres.
A Puffy Planet
A variety of different elements and spectral lines have been used to study exoplanet atmospheres: Lyman-α and Hα (both hydrogen lines) are most common, as well as other heavier elements like carbon and oxygen. It wasn’t until very recently that we are also able to detect and use another element: helium. Ironically, helium is the second-most abundant element in the universe, but it is very hard to detect since, being a noble gas, it’s difficult to excite enough to give off any absorption or emission. In 2018, astronomers detected helium for the first time in the exoplanet WASP-107b, a sub-saturn orbiting a star a bit smaller and cooler than our Sun. Now in this paper, those same authors are using the helium lines in this planet to learn more about its extended atmosphere.
Studying the chemical composition of exoplanet atmospheres can give us a lot of clues about their conditions and potential habitability. The authors of today’s paper are specifically interested in studying atmospheric escape, or how gases from the planet’s atmosphere gain enough energy to overcome the gravitational pull of the planet and escape into space. This can cause the planet to lose its “safety blanket” shielding it from harsh solar radiation, cosmic rays, potential asteroid impacts, and other life-threatening matter and radiation. This is especially evident in planets that orbit very close to their host star where radiation and stellar wind can cause the atmosphere to evaporate away.
Let There Be Light!
But studying this is no piece of cake: we can’t actually point a telescope at WASP-107b and take a picture of its atmosphere. In fact, there have only been a handful of directly imaged exoplanets, as the glare from a host star far outshines any light coming from or reflected by a planet. Instead, we rely on obtaining spectra of the star during the exoplanet’s transit across its surface. When WASP-107b passes in front of its host star, a small portion of the light emitted from the star passes through the planet’s atmosphere and either penetrates it or gets absorbed, depending on the wavelength. Using a technique called transmission spectroscopy, we can figure out what elements are present in the atmosphere by looking at what wavelengths of light have been absorbed and then study those absorption lines in more detail. By measuring the spectra both in transit (when the planet is passing in front of the star) and out of transit, astronomers can calculate the difference between these spectra and see which spectral lines have excess absorption. If an element is present in the exoplanet’s atmosphere, the measured spectral line should have a greater depth while the planet is in transit.
Using the Keck NIRSPEC spectrograph, the authors observed the absorption feature of WASP-107b at 10833Å, the wavelength around three helium lines.
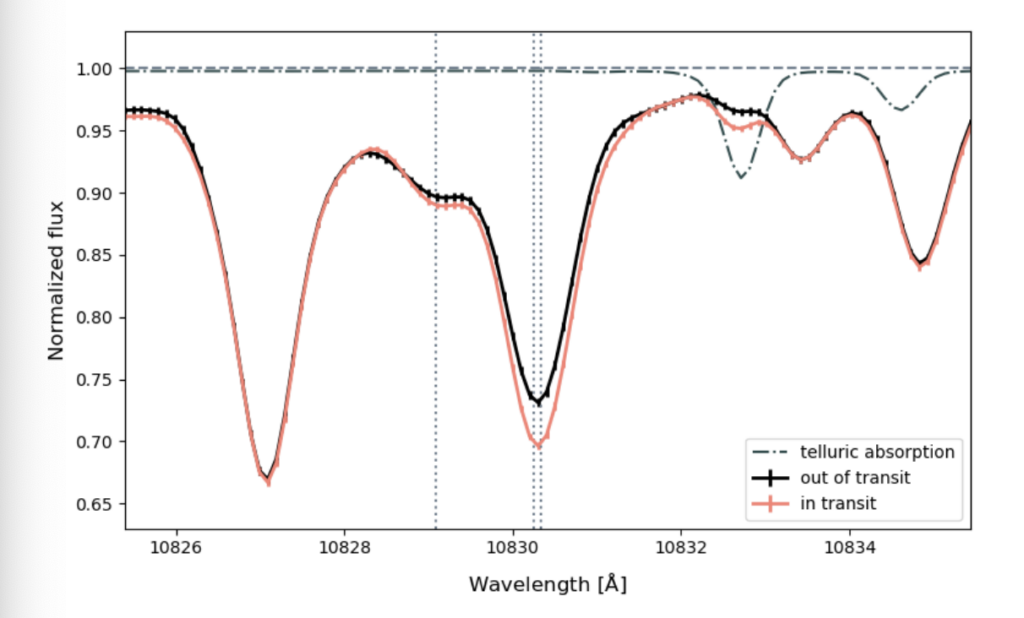
Figure 1 shows the resulting spectrum. The black line is the average spectrum from points measured when WASP-107b was outside transit, and the red line is from points measured during its transit. The absorption feature around 10833Å (where the two dotted grey lines are) is deeper for the in transit spectrum than the out of transit one, indicating that there is excess absorption of helium during transit and hence is present in the atmosphere of WASP-107b.
The Same Procedure…Yields a Different Answer?
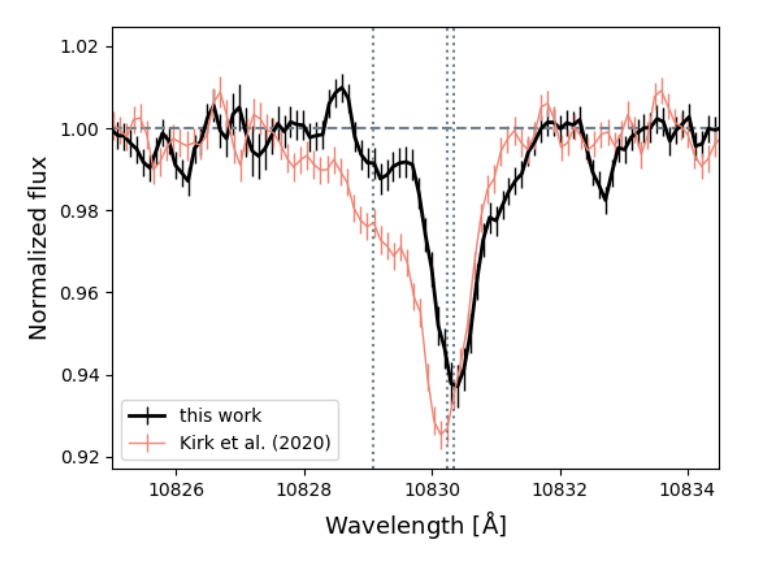
The ratio between the in and out of transit spectra is shown in Figure 2, in black. The red line shows the same ratio, but using data from another group (Kirk et al.) that took data on WASP-107b a few months later. Surprisingly, these spectra don’t match: the black spectrum has a shallower dip and looks redshifted in comparison. This is strange, because one would think that if you take a spectrum of the same planet, it would look very similar no matter when you got your data, since nothing about the planet or star should radically change between a few months.
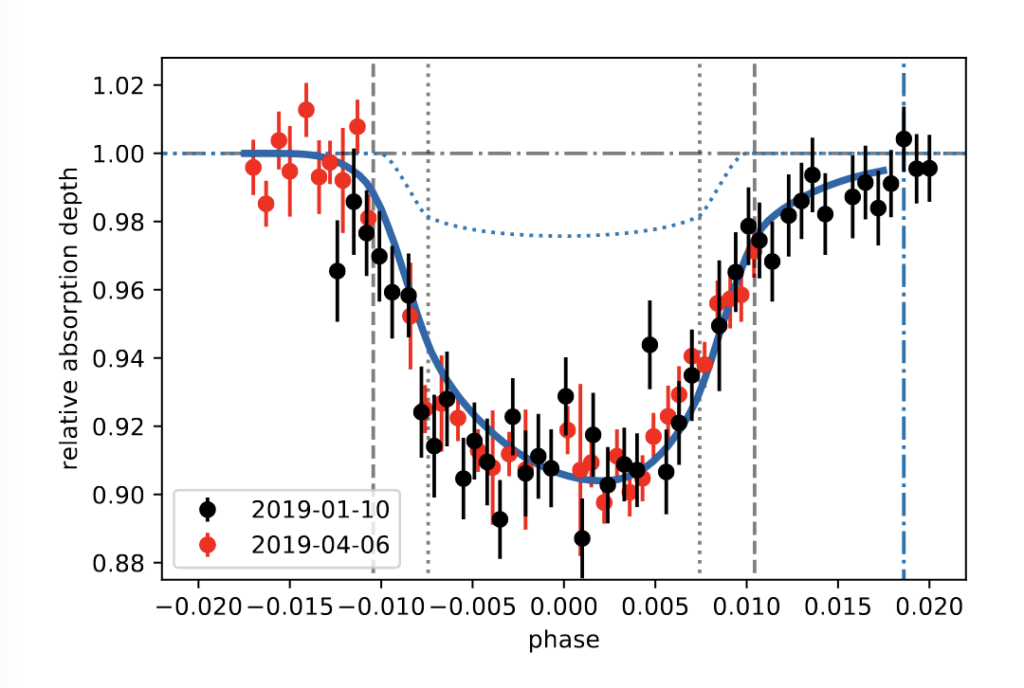
So the authors dug deeper into this question and calculated the excess helium absorption spectrum integrated over wavelengths around the absorption feature. Now, instead of seeing the flux from the star over different wavelengths, we can see how the excess helium depth changes over time as WASP-107b moves across the surface of its host. This is shown in Figure 3. The black points are data taken by the authors and the red points are data from Kirk et al., normalized so that their transit depths match. There are two important things to note about this figure: 1) the light curve is asymmetric and tapers off slower after transit than before transit, and 2) the authors’ data extends further into the post transit regime than Kirk et al.’s, which stops just as the planet has finished transiting.
What’s in a Tail?
The fact that there’s still absorption after WASP-107b has finished transiting leads the authors to infer that this planet actually has a tail: part of its atmosphere trails out behind it like a comet and escapes into space, causing us to measure the helium in its atmosphere even after WASP-107b is no longer in front of its host star! The existence of planetary tails has actually been predicted by hydrodynamical simulations–in fact, another group modeled WASP-107b’s atmosphere and the resulting lightcurve is shown as a solid blue line in Figure 3. It matches very closely with the data from this paper, corroborating the idea that WASP-107b has a planetary tail. They calculate that this tail extends out to 7 times WASP-107b’s radius, or roughly twice its Roche lobe radius, the longest ever observed at 10830Å.
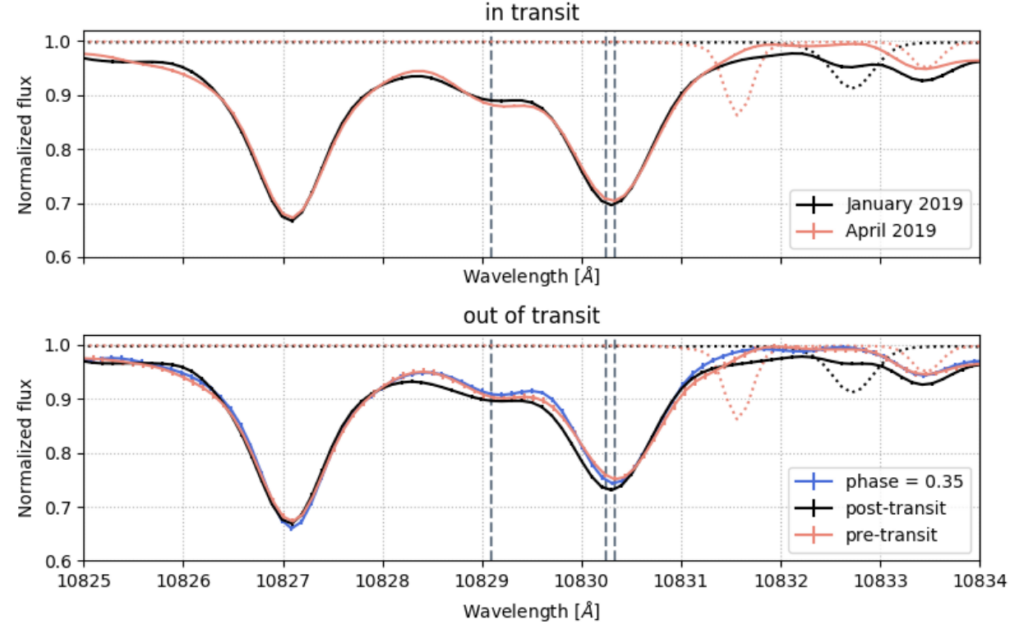
In addition, if we look at Figure 4, we see that the in transit spectra from this paper and from Kirk et al. is quite similar, but the out of transit spectra are different. The helium absorption is deeper post-transit than either pre-transit or when the planet was nowhere near close to transiting. This indicates that WASP-107b’s has an extended tail on only one side of the planet. Since the in-transit spectra are quite similar, any differences between the authors’ data and Kirk et al.’s would come from the out of transit spectra, and we saw in Figure 3 that Kirk et al.’s data didn’t extend into the post-transit where the author’s observed WASP-107b’s long tail, which could explain the discrepancy between their spectra from Figure 2.
Though the authors also considered other potential reasons for why their spectrum differs from Kirk et al.’s, like WASP-107b passing over active or quiet regions of its star at different times, in the end they concluded that a post-transit tail is the best explanation. This result is an exciting step toward shedding light on how atmospheric escape works and what kinds of planets are more susceptible to it, and hopefully with more observations, we’ll be able to explore the effect of stellar winds on exoplanet atmospheres.
Astrobite edited by Huei Sears
Featured image credit: Nature Astronomy, https://www.nature.com/articles/d41586-018-04969-6